
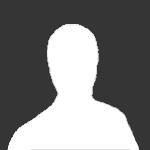
Widdekind
-
Posts
1121 -
Joined
-
Last visited
Content Type
Profiles
Forums
Events
Posts posted by Widdekind
-
-
(Thanks for the responses.)
Typically, interacting Wave Functions do not "collapse", but become "entangled", entering a single state for all of the entangled particles. "Measurement" destroys this singular entangled state, causes all of the entangled Wave Functions to collapse, and, thereby, puts all of the particles into individually separate states. Would not that increase the system's entropy (making more states available to the ensemble) ?
Virtually every time two particles interact and aren't measured, they become entangled...When two particles interact, there is no Wave Function Collapse. But, when a particle interacts with a 'measuring device', the measurement has a definite outcome: the particle's Wave Function "collapses" into a pure state, of whatever the device measures.
Marc Lange. An Introduction to the Philosophy of Physics, pp. 259,297.
0 -
I found these references, seemingly suggesting, that nucleons "clique" together, inside nuclei, w/ pions apparently representing "thermal collisional" excitations (my words), which I would guess come from "stressing & stretching" the "glue" linking & locking quark-triplets together, inside nucleons:
In the nucleus, the scattering can happen on a nucleon cluster, and the scattered electron may acquire a larger momentum than on a free nucleon...If one supplies energy to a normal nucleus, it heats up, and emits nucleons or small nuclei (mainly alpha clusters [math]\left( \alpha = He^{2+} \right)[/math]), just as a liquid droplet evaporates atoms or molecules. If, however, one confines the material, increasing the energy supplied leads [instead] to the excitation of internal degrees of freedom. In a molecular gas, these are rotational & vibrational excitations. In nuclei, nucleons can be excited into [math]\Delta(1232)[/math] [spin] resonances, or to still higher nucleon states. We have called the mish-mash of nucleons & pions, where are then created by decays, Hadronic Matter.
Bogdan Povh, Klaus Rith. Particles & Nuclei, pp. 93,321.
The Wave [Function] of an alpha particle, within a heavy radioactive nucleus, has a tiny [diffuse] "tail" that extends well outside the nucleus. Because of this remote bit of Wave [Function], the alpha particle has a certain probability of springing through the force barrier that would otherwise hold it within the nucleus, whereupon it flies away, to signal an alpha-decay event. This is the tunneling phenomenon... explained in the quantum world through the wave nature of matter, and the link between waves & probability.K.W.Ford. The Quantum World, pg. 203.
At normal nuclear densities, each nucleon occupies a volume of about 6 fm3, whereas the actual volume of a nucleon itself is only about a tenth of this [0.6 fm3]. If one, then, were to compress a cold nucleus (T=0) to ten times its usual density, the individual nucleons would overlap, and cease to exist as individual particles.Bogdan Povh, Klaus Rith. Particles & Nuclei, pg. 321.
Virtual mesons surround ("clothe") the Dirac ("bare") nucleon... Nucleons are composed primarily of three quarks, the proton has the composition (uud), the neutron (udd), where u stands of an up quark, and d for a down quark. Nucleons contain not just one point particle and a meson cloud; three point particles reside there. The interaction among the quarks is transmitted by gluons; the force is weak at short distances ([math]\leq 0.1 fm[/math]) and strong at large ones ([math]\geq 0.5 fm[/math])... The [virtual] mesons are an effective means of describing "large" distance hadronic structure. Pions are the lightest mesons, thus they account for the outermost part of the structure, and are, therefore, the most important ones to consider in addition to the quarks... A number of "bag" models have been constructed; some of the more successful ones include a pion cloud in addition to the quarks, to explain the structure of the nucleon. In such a picture, a photon interacts not only with the core (bare proton or [three] quarks), but also with the surrounding meson cloud. Since the pions do not leave the nucleon, and have to return, they can only go about half the pion Compton Wavelength. The radius of the nucleons, consequently, is expected to be about [math]\hbar / 2 m_{\pi} c[/math], or about 0.7 fm. In this model, which can account for the static properties of both the proton & neutron, the quarks and the pion cloud contribute to the magnetic moment.Ernest M. Henley, Alejandro Garcia. Subatomic Physics, pp. 153-154.
We describe the proton as three constituent, or valence, quarks ([math]u_v u_v d_v[/math]), accompanied by many quark-antiquark pairs ([math]u_s u_s, d_s d_s, s_s s_s[/math], and so on). These are known as "sea" quarks. If we picture them as being radiated by the valence quarks, then, as a first approximation, we may assume that the three lightest flavor quarks (u,d,s) occur in the "sea", with roughly the same frequency & momentum distribution, and neglect the heavier flavor quark pairs ([math]c_s c_s[/math], and so on).Francis Halzen, Alan D. Martin. Quarks and Leptons, 198.
Complicated processes are going on inside [nucleons] -- valence quarks emitting virtual gluons, gluons producing quark-antiquark pairs, [and these] 'sea' quarks recombining, and so on... all this frenzied activity conserves [electric] charge.J.D.Griffiths. Introduction to Elementary Particles, pg. 320.
EDIT: These last two sources seemingly suggest, that pions represent a radial, quasi-periodic, "breather mode", expansion of core [constituent / valence / structural] quarks:
[math](d u u) \rightarrow (d u) u[/math][up quarkinprotongets "quantum kick" (of some sort)...][math]\rightarrow (d u) + g + u[/math][up quark"puffs out" radially, stretchinggluonbonds, which thusly "ooze more glue"][math]\rightarrow (d u) + (d \bar{d}) + u[/math][up quark"swells" further still, stretchinggluonbonds until they break, "ripping" intoquark-antiquarkpairs][math]\rightarrow ((d u) + d) + (\bar{d} u)[/math][up quark's expansion "stalls out", as itHadronizeswith theantidown antiquark, whilstdown quark, left behind at smaller radii,Hadronizeswith "spectator"structural quarks, to form aneutron][math]\rightarrow (d u) + (d \bar{d}) + u[/math][up quarkbegins being brought back inwards, in "infall"...][math]\rightarrow (d u) + g + u[/math][up quarkcontinues "deflating",quark-antiquarkrecombine intogluonbond][math]\rightarrow (d u) u[/math][quasi-stableprotonhas reformed]This "Cepheid Variable pulsating breather mode" model, of virtual pion production in protons, seems consistent w/ claims, that the proton spends ~80% of its time being "bare", and ~20% of its time "dissociated" into neutron + pion [looking for the book every which where].
EDIT to EDIT: According to I.S.Hughes' Elementary Particles (3rd. ed.), p.51, " part of the time the proton exists as a neutron plus a [math]\pi^{+} meson[/math], [math]p \rightleftharpoons n + \pi^{+}[/math] ", which orbits the central nucleon in an L=1 p-state. Given that gluons have S=1, the emission of a gluon, by a quark (S=1/2), Conservation of Angular Momentum must imply that the quark spin-flips; and, that, when the gluon bond breaks, the resulting quark-antiquark pair produced must be "born" spin-parallel:
[math]p^{+} = (d \downarrow u \uparrow u \uparrow) \rightarrow (d \downarrow u \uparrow) \; u \uparrow[/math][math]\rightarrow (d \downarrow u \uparrow) + g \uparrow + \; u \downarrow[/math][math]\rightarrow (d \downarrow u \uparrow) + (d \uparrow \bar{d} \uparrow) + u \downarrow[/math][math]\rightarrow \left( (d \downarrow u \uparrow) + d \uparrow \right) + (\bar{d} \uparrow u \downarrow)[/math][math]\rightarrow \left( d \downarrow u \uparrow d \downarrow \right) + \pi^{+}_{l=1}[/math][the spin-updown quark, from the torn tendon ofglue,Hadronizeswithspectator quarksin the core, but must spin-flip to spin-down, to align with its new-found fellowdown quark. That spin-flip can account for the "Angular Momentumboost" (L=0 to L=1) given to the newlyHadronizedpion.][math]= n + \pi^{+}_p[/math][math]\rightarrow \left( (d \downarrow u \uparrow) + d \uparrow \right) + (\bar{d} \uparrow u \downarrow)[/math][math]\rightarrow (d \downarrow u \uparrow) + (d \uparrow \bar{d} \uparrow) + u \downarrow[/math][math]\rightarrow (d \downarrow u \uparrow) + g \uparrow + \; u \downarrow[/math][math]\rightarrow (d \downarrow u \uparrow u \uparrow) = p^{+}[/math]In-so-far as any kind of crude comparison can be construed, between the L=1 p-state pion, and the corresponding Hydrogenic Wave Function (L=1, m=1 [?]), then the Wave Function of the "spun up" pion perhaps takes on a toroidal, donut-like shape, rotating around the neutron at the center. According to Hughes (ibid.), this simple model predicts that protons spend a time-fraction x=0.7 in the "bare" proton state, and the rest of the time in the "dressed" neutron + pion state. Repeating the process for neutrons ([math]n \leftrightharpoons p + \pi^{-}[/math]), yields a bare-state fraction x=0.76. If meaningful, this might imply, that the lighter, and more electrostatically repulsive, up quarks, in protons, are more likely to "poof out", into the "pion breather" mode, than the lighter & less repulsive down quarks, in neutrons.
Note that the "splitting" of gluons into quark-antiquark pairs seems superficially similar to the "splitting" of photons into electron-positron pairs.
0 -
Are there any known examples, of interactions involving Wave Function Collapse, which conserve (interacting) particle number (or, is there always an "accompanying" annihilation / creation event, of a whole quanta of Wave Function) ?
0 -
If quantum mechanical "measurement" represents an irreversible alteration, to the Wave Function, of a quantum system (causing its "collapse" into a singular state)...
then doesn't QM measurement resemble Entropy, which irreversibly increases with time? What other physical processes, or quantities, are also "irreversible" ??
(Thanks in advance)
0 -
I understand, that you cannot send meaningful information on individual photons, since their behavior is random. But, what about the patterns produced by ensembles of photons ("fringes" vs. "heaps"), passing through the slits ? Measurement / observation causes Wave Function Collapse, which changes the statistical distribution of detector-screen hits to change, from "fringes mode" to "heaps mode". If so, using a conventional "carrier beam signal" (of suitably entangled photons), and assuming that the lengths of the "legs" were (sufficiently) the same, each user could cause, or cause not, the collapse of the photons' Wave Functions, switching the system from "fringes" to "heaps", which could encode information (if the carrier beam was continuously maintained, and by pre-arranged agreement). What am I missing ?
There are effectively three different levels of operation in a quantum measurement. The first consists of the way the measuring apparatus is set up (e.g. which components of polarization are being measured). The second level is the statistical result that is obtained after a large number of measurements have been made (e.g. how many photons emerge in each channel). The third is the result actually obtained in a particular, individual measurement. As far as this last is concerned... this is completely random & unpredictable.. As far as measurements on an individual photon pair are concerned, the results of these third-level processes are random & unpredictable, whatever the setting of the apparatus. The second-level statistical predictions are affected by first-level changes in a way that can predicted by quantum theory.A.Rae. Quantum Physics, pp. 56-57.
0 -
We wish to produce a mono-chromatic beam, with momentum p = 20 GeV [[math]\lambda_C \equiv h / m c = 6 \times 10^{-17} m[/math]], and momentum spread [math]\Delta p / p[/math] of 1% [[math]\Delta x \equiv \hbar / \Delta p = 10^{-15}m[/math]]. The beam is 2 mm wide.
Alessandro Bettini. Introduction to Elementary Particle Physics, pg. 82.
LEP was a circular machine, in a tunnel 27 km long. This is testament to the problems with lightweight electrons & positrons, traveling around in circles, that such a distance is needed to enable them to reach 100 GeV without wasting too much energy in [synchrotron] radiation. To reach energies of several hundred GeV, in circular orbits, would require distances of hundreds of kilometers, which are out of the question. This is why linear colliders are planned for the longer-term future.The idea is to have one linear accelerator of electrons, and another accelerating positrons... To have a decent chance of a collision in a [head-on] linear accelerator, where the beams meet only once, requires high intensity beams that are less than a micron (10-6m) across.
Frank Close. [VSI] Particle Physics, pg. 57.
When the LEP began running in the 1990s [100 GeV], needle-like bunches of electrons & positrons would pass through each other, at the heart of the detector, every 22 microseconds (22 millionths of a second) [[math]22 \mu s \times c = 7 km[/math]]. Even though there were some million million particles in each bunch, the particles were thinly dispersed, so interactions between them were rare. An interesting collision, or 'event', only occurred about once every 40 times or so the bunches crossed.
ibid., pg. 59.
At the Large Hadron Collider (LHC)... the ATLAS detector will be five stories high (20 m), and yet able to measure particle tracks to a precision of 0.01 mm [10-5m].
ibid., pg. 77.
We want to procure a source of protons, and accelerate them to... about 99.999999% the speed of light. Then we need to make a beam of these protons, no wider than the width of a human hair [~100 microns], and guide the beam in a circular path of a day or so, during which time a proton will travel 26 billion km...The LHC beam... is comprised of bunches of protons, with each bunch separated by no less than 7.6 m... This is the distance between adjacent waves in the accelerating electric field [40 MHz]. Of course, it is possible for adjacent bunches to be farther apart than this. If not every wave is filled with protons, adjacent bunches could be multiples of this distance, depending on how many waves are skipped...
An individual bunch [of protons] in the LHC includes about 100 billion (1011) protons. The actual shape of each bunch has a passing resemblance to a stick of uncooked spaghetti, although it is about 0.3 m (1 foot) long, and the width is less than a millimeter. There will be 2,808 bunches of protons orbiting in each direction, and aimed & focused to collide at four points around the LHC's perimeter. So, except for the actual beam width being about a hundred times smaller than a piece of spaghetti [1% of 1 mm], you can get a pretty good visualization, of the LHC's beam, as [being] about 3,000 pieces of uncooked spaghetti, each separated by about 7.6 m (25 feet), orbiting at 99.999999% the speed of light, in an orbit that is 27 km (17 miles) around. if you do the math, you find that about 3,800 lengths are needed to fill up the entire orbit. So, if there are 2,808 bunches, the entire accelerator is not filled. You have a concentrated group of bunches, each separated by the 7.6 m (25 feet), followed by a relatively long gap. This gap has many uses. Note that "long" is a relative term, and in the ballpark of a millionth of a second (microsec). This exceedingly brief time, during which there are no protons in a detector, is used for the detector to recover & reset itself.
Don Lincoln. The Quantum Frontier: The Large Hadron Collider, pp. 67,93.
The method proposed by Fermi is based on the assumption, that in the collision of high-energy nucleons, the energy appears simultaneously in a small volume, and that both nucleons come to rest, in the center-of-mass system.Because of the Lorentz Contraction, this volume is not spherical, but is compressed in the direction of motion of the nucleons prior to the collision. The amount of compression is given by the well-known factor:
[math]\sqrt{1 - \frac{v^2}{c^2}} = \frac{M c^2}{E'} = \gamma^{-1}[/math]where M is the mass of the nucleon, and E' its energy, in the center-of-mass system... The volume of the region in which energy appears is (order of magnitude):
[math]\Omega \approx \frac{M c^2}{E'} \left( \frac{\hbar}{\mu c} \right)^3 = \frac{\lambda_{C,\pi}^3}{\gamma}[/math]since [math]\hbar / \mu c[/math] ([math] \mu[/math] is the [pi-]meson mass) is of the order of the interaction range.
L.D.Landau & Ya.Smorodinsky. Lectures on Nuclear Theory, pg. 105.
0 -
Note the phrase virtual process in your quote.
(Thanks for pointing that out.)
Virtual pions are "off-mass shell" ? Actual pions would not help bind nuclei together, for fusion effects ?
0 -
How big are Collider Beams, used in High Energy collisions ? For example, in Deep Inelastic Scattering experiments, beaming Leptons (electrons, neutrinos) at nucleons, how physically big & broad are the Leptonic Wave Functions ?
0 -
"Quarks never appear in isolation. This process of Hadronization occurs before quarks formed in high-energy collisions can interact in any other way. The only exception is the top quark, which may decay before it Hadronizes" (ISBN 9781155255507, pg. 50).
Thanks for all the responses
0 -
A pion is a Meson, a "middle-weight" combo of a quark & antiquark, whose mass-energy equivalent is ~140 MeV (~1/7th of nucleon mass-energies). They flit between nucleons, in a nucleus, and bind them all together, thereby accounting for the Strong Force, to my understanding. Their Wave Functions are apparently more extended, distended, or delocalized, than those of the nucleons themselves, which are typically spread out over ~1 fm. Thus, the "pion cloud" has a "tail" which extends out well beyond the "surface" of the nucleons to which they are bound.
0 -
According to the book Biocentrism (pp. 78ff), by Robert Lanza, by means of a continuous beam of Quantum Entangled, separated photons (emerging from a BBO crystal), the presence or absence of Interference Fringes, observed on one side of the set-up, can be instantaneously determined, by inserting / withdrawing a scrambler, from the beam on the second side of the set-up. In essence, the person on the second side plays "handsies in front of the projector screen" with their half of the split beam. Assuming that (1) such a split beam was sent to two remote locations (S & P) conventionally, at the speed of light; and, (2) that said split beam was continuously maintained, from the central source; then, (3) could not binary bits of information, be instantaneously sent, from P to S, based upon the presence / absence, of interference fringes, observed at S ??? Such a set up would allow a "central HQ" to feed a "laser umbilical" out to "soldiers" in the field, who would then be able to "cross talk", between themselves, instantaneously (in binary).
0 -
Nucleons are apparently immersed in a "pion cloud", of extended, delocalized, diffuse pions. And, pions apparently produce the Yukawa Potential, to mediate the Strong Nuclear Force. What role do pions play, in nuclear fusion processes ? Could you create some sort of "pion catalyzed fusion", along the lines of [math]p^{+} + p^{+} + \pi \rightarrow He^{2+}:\pi \rightarrow He^{2+} + \pi[/math] ? Could you "pre-treat" fusion fuels, by bombarding the protons in order to "stress" their gluon fields, thereby (electro- or neutrino-)producing quark/antiquark pairs (as gluon bonds break), which could "hadronize" into pions, and make the protons "pion rich" and "extra (Strong Force) sticky" ???
Anthony W. Thomas, Wolfram Weise. The Structure of the Nucleon:
At low energy, the nucleon an be viewed, as a system of three constituent quarks, surrounded by an interacting cloud of pions.(pg. 192)
The role of the pion cloud of the nucleon is vital... The dominant long-range structure of the proton, is given by the virtual process [math] p \rightarrow n \pi^{+}[/math]; and, in a simple valence picture of the nucleon and the pion, this Fock component of the wave-function involves only [math]\bar{d}[/math] quarks, not [math]\bar{u}[/math].
(pg. 94)
Pions are also an important feature of nucleon structure itself. When probing the nucleon with long-wavelength, electro-weak fields, a substantial part of the response comes from the pion cloud, the "soft" surface of the nucleon.
(pg. 202)
The structure of a physical hadron will consist of a valence quark core "dressed" by a cloud of pions.
(pg. 236)
0 -
Asymptotic Freedom -- Quarks are like Tether-Balls, "feeling free off the fist", but bound back to the pole after a few feet (???)
According to the Bag Model, inside Hadrons, quarks are kept confined by a bag-like "skin" of "sticky slime". That "glue" is concentrated at the surface "skin" of the Hadron (r ~ 10-15m). When high-energy Leptons are beamed at, and into, Hadrons, they stream through that "sticky slime skin", probably (???) w/o interacting with those gluons, since Leptons are "color blind", and do not interact via the Strong (Color) Force. Once inside the Hadron, the Leptons interact with the constituent 'structural' quarks, causing their Wave Functions to collapse, or localize, into little (apparently) point-particles (r < 10-18m). At first, before those collapsed constituent quarks can careen out into the "sticky slime skin" of the gluon field, they scatter the incident Leptons like free particles.
Now, at low Lepton energies, when gluon bonds begin to break, perhaps (???) a little like taughtened taffy thinning in the middle, those gluons can beget more gluons, to help hold together the tendon-like bond. In this "slow stretch" mode, the Lepton's incident energy can be absorbed into the "glue", perhaps (???) a little like kevlar bringing a bullet to a soft stop. But, at high Lepton energies, quarks are thrust through the "sticky slime" far too fast for that "re-forming effect", of the gluons' broken bonds, to take place. Thus, the threads of "sticky slime" simply "snap", before any incident Kinetic Energy can be absorbed into those bonds -- perhaps (???) a little like a swiftly speeding sniper bullet penetrating a kevlar coat. Thereby, harder & harder hit quarks act as if they are "entrained" with less & less glue, and so look like lighter & lighter (point) particles.
To resolve the interior structures of nucleons (r ~ 10-15m), Leptons must have high energy (l < 10-15m, E > 1 GeV). Thus, nucleons (m c2 ~ 1 GeV) can disintegrate under such circumstances, shattering into a "scree spray" of naked quarks, antiquarks, & gluons, each carrying only a tiny fraction of the original nucleon's momentum. However, such collisions are often quasi-elastic, so that the incident Lepton only transfers a tiny fraction, of its incident energy, into the nucleon. In some sense, the Lepton's Wave Function, then, "flows through", and only "ruffles", the nucleonic Wave Functions, of the three structural quark cores, and all of their entrained gluons.
References:
G. Aubrecht. Quarks, Quasars, & Quandries, pp. 68-72.
We talked about electron-positron annihilation, into Hadrons, proceeding through the formation of a quark-antiquark pair. The quarks materialize into Hadrons... which remember the direction of their parent quarks [Conservation of Momentum ???]. That gives us two-jet events.In much the same way, now that we have invented gluons interacting w/ the quarks, we may imagine that sometimes one of the outgoing quarks radiates a gluon:
electron-positron --> quark + antiquark --> (quark + gluon) + antiquark...When that happens, I expect my two-jet event to change into a three-jet event. One quark jet splits into a quark jet and a gluon jet.
At high energies, in electron-positron annihilations, three-jet events are quite common... [Often] you can see one fully developed jet, and two smaller jets. The fully developed jet may represent debris from the quark. Then, the smaller jets are the offspring of the antiquark & gluon. The frequency at which these events are seen, and the detected properties of the events, are all consistent with the idea that the mechanism for generating them really is a quark, an antiquark, and a gluon, in the semifinal state before the Hadrons materialize [Hadronization].
Now I want to talk about [vacuum] polarization effects, & the effective [colour] charge of the quarks. There will be similar screening effects to the one we discussed for [quantum] electrodynamics... Just as before, if I ask, "at a certain radius, how much redness lies w/in a circle [sphere]?", the result will be less than the redness of the test [colour] charge, b/c some of it is screened out, or canceled... There is color charge screening in this case, which tells you that the effective [colour] charge tends to become larger, as you probe on shorter distance scales. This is entirely analogous to what we saw in QED.
The difference, in this case, is that there is something else that can happen, b/c gluons carry color [charge]. B/c the gluons carry color [charge], quarks can Camouflage themselves, and hide their color... [imagine a] red quark. We now send some emissary in, to say, "hello, are you red?" While our emissary is on the way in to ask whether this is a red quark, the quark can fluctuate, quantum mechanically, into a quark and a gluon. And, if it chooses to, it can fluctuate into a green quark, and a red-antigreen gluon. The red-antigreen gluon goes out, and takes a walk, in quantum mechanics space...
Our probe arrives, and says, "hello, are you red?" And the quark says, "no, I'm green, go away." So, b/c of the fluctuations made possible by the fact that gluons can carry color, you find, if you look too closely, less red charge than you thought was there. In order to see the full red charge, you've got to look on a bigger scale, the scale of the promenade of the gluons.
We have two effects going on: one, the normal screening effect as in [quantum] electrodynamics; the second, the Camouflage effect, made possible b/c, unlike photons, which don't have an electric charge, the gluons do have a color charge.
There's a competition, between these two effects, and in the theory we believe to be true, QCD, Camouflage wins. The consequence of this, is that the Strong [Colour] Force, as measured by the effective color charge, becomes weaker and weaker at short distances. If you look closer and closer, you find that the strong [colour] charge is getting tinier and tinier. What this means, is that, for practical purposes, if you find quarks close together, in a small space inside a bubble or a little balloon or bag, they behave almost as independent particles. B/c of this Camouflage effect, as long as the quarks remain close together, each one hardly feels the color charge of the others.
On the other hand, if you try to separate two quarks by a large distance, then each is able to see more clearly the full charge of the neighboring (but no longer very close) quark. And, so, the Strong [Colour] Force becomes more formidable, as you go to large distances. We believe that this effect, properly implemented, is responsible for the fact, that we can talk about the quarks as being quasi-free particles w/in protons, but we can't extract the quarks from the protons. The net antiscreening of color charge gives us the possibility of understanding that apparent paradox.
Fig. 3 --Apparent relation, ofGluondensity, to gradient ofQuarkdensity, seemingly suggests(???)some sort of "self-consistent" solution, and/or thatQuarkmomentum is associated w/Gluonemission. This picture apparently purports, thatNucleonsare a little like "gluebubbles". The "sticky slime" slathers the surfaces ofquarks, "constricting" them radially inwards, with powerful implosive pressure.Fig. 5 --ByCamouflage,quarksexpel theircolor charge, thereby "blanching" and becomingcolor neutral(White). In crude conception,quarks"blanch" by "oozing" a shrouding "Camouflagingcocoon" cladding of "sticky slime"glue, composed of agluon triplet. Eachgluonin thetripletcarries, quantum mechanically, 1/3rdof the emanatingquark's color charge, combined with 1 of the 3anticolors. Thus, eachgluon triplet, in sum, carries 1color, whilst beinganticolor antineutral(Black). Then, each "blanched"color neutralquark(White) attracts the "blackened"anticolor antineutralgluon triplet(Black) that it emanated(White <---> Black). Meanwhile, those 3gluon triplets, each carrying, quantum mechanically, themonochromatic color charge, of their parentquarks, are themselves mutually attracted & bound, by theStrong (Color) Force, thereby becoming, in combination,color neutral(White). Thus, inLepto-productioncollisions, the incidentLeptonsinteractElectromagneticallywith theCamouflagedconstituent quark cores, which interactChromodynamicallywith their ownCamouflaging"cocoon cladding" ofcoloredglue, which "sticky slime shrouding shells" mutually interact, againChromodynamically:Lepton<---EM--->quarks<---CD--->owngluons<---CD--->others'gluonsThis "loose linkage" may act as a kind of "shock absorber", complicating the dynamics ofLepto-productioncollisional interactions.Andrew Watson. The Quantum Quark, pp. 285-287.
How to reconcile these quark model masses, w/ the other set of oft-quoted quark mass values? In Chapter 4, the up & down quarks were ascribed masses of 4 MeV & 7 MeV, respectively, very different from the 300 MeV or so for the constituent quark masses. These smaller values, extracted from pion-decay data, are termed current quark masses... A constituent quark is a current quark surrounded by a gluon cloud, and it's this cloud that carries most of the mass, and which gives rise to the difference between the two sets of mass values...The quark mass depends on energy. In this way, quark masses are not fixed, but are "running" masses that decrease as the energy is increased... For three-jet production, in electron-positron collisions, Marti i Garcia and his colleagues deduced a value of 2.67 +/- 0.50 GeV for the b quark mass. Their figure, extracted at an energy of 91 GeV, the mass of the Z boson, is just 60% of the quoted value of the b quark mass [4.5 GeV] at 1/20 of the energy [4.5 GeV], based on studies of the Upsilon meson [Bottomonium in excited Spin state (S=1)]. This fall in b quark mass with increasing energy is in line with the running mass prediction of QCD.
Books LLC. Quarks: Quark, Strange Quark, Top Quark, Isospin, Down Quark, Up Quark, Bottom Quark, Hypercharge, Charm Quark, Baryon Number.
Part of the effects of virtual quarks & virtual gluons, in the 'sea', can be assigned to one quark so well, that the term 'constituent' quark ['structural quark'] seems appropriate. According to the Feynman Diagrams, constituent quarks seem to be 'dressed' current quarks, i.e., current quarks surrounded by a cloud of virtual quarks & gluons. This [gluon] cloud, in the end, explains the large [effective] constituent quark masses. The effective quark mass is called the constituent quark mass. Hadrons consist of "glued [together]" constituent quarks.(pp. 17-18)
Current quarks (also called naked quarks) are defined as the constituent quark cores (constituent quarks with no covering [of glue]) of a valence quark. If, in one constituent quark, the current quark is hit inside the covering with large force [high energy], it accelerates through the covering, and leaves it behind. In addition, current quarks possess asymptotic freedom (w/in the perturbation theory described limits). The mass of the current quarks carries the designation current quark mass.
(pg. 19)
The current quark mass is also called the mass of the 'naked' quarks. The mass of the current quark is reduced by the term of the constituent quark covering mass... The current quark masses, of the light current quarks, are much smaller than the constituent quark masses. [The] reason for this is the missing of the mass of the constituent quark covering [associated "glue"]...
Definition: The current quark mass [~5 MeV] means the mass of the constituent quark [~300 MeV], reduced by the mass of the respective constituent quark covering [adhering "glue"].
(pp. 20-21)
Two terms are used in referring to a quark's mass: current quark mass refers to the mass of a quark by itself ["naked" quark], while constituent quark mass refers to the current quark mass plus the gluon particle field surrounding the quark ["dressed" quark]. These masses typically have very different values. Most of a Hadron's mass comes from the gluons that bind the constituent quarks together, rather than from the quarks themselves. While gluons are inherently massless, they possess energy -- more specifically, Quantum Chromodynamics Binding Energy (QCBE) -- and it is this that contributes so greatly to the overall mass of the Hadron. For example, a proton has a mass of approximately 938 MeV/c2, of which the rest of masses of its three valence quarks only contribute about 11 MeV/c2; much of the remainder can be attributed to the gluons' QCBE.
(pg. 49)
Since gluons carry color charge, they themselves are able to emit & absorb other gluons. This causes asymptotic freedom: as quarks come closer to each other, the Chromodynamic Binding Force between them weakens. Conversely, as the distance between quarks increases, the binding force strengthens. The color field becomes stressed, much as an elastic band is stressed when stretched, and more gluons of the appropriate color are spontaneously created to strengthen the field ["slow stretch" mode]. Above a certain energy threshold, pairs of quarks & antiquarks are created ["fast stretch" tearing mode]. These pairs bind with the quarks being separated, causing new Hadrons to form. This phenomenon is known as color confinement: quarks never appear in isolation. This process of Hadronization occurs before quarks formed in high-energy collisions are able to interact in any other way. The only exception is the top quark, which may decay before it Hadronizes.
(pg. 50)
0 -
"Best of Both Worlds" Resolution (???) -- Unperturbed Quark Wave Functions are extended [~10-15m], but collapse into quasi-structureless points [< 10-18m] upon Perturbation
(1) Quark Wave Functions collapse upon perturbation by impinging Electrons / Neutrinos
According to Robert Lanza's Biocentrism:
No small object actually assumes a particular place, or motion, until its Wave Function collapses. What accomplishes this collapse? Messing with it in any way. Hitting it with a bit of light, in order to "take its picture", would instantly do the job.(pp. 50-51)
(2) Immediately after perturbation impact, Nucleons are comprised of 3 point-like [Valence] Quarks in a "sea" of Gluons & Quark/Antiquark pairs [produced upon Gluon bonds breaking]
According to F.E.Close's An Introduction to Quarks & Partons:
pion production begins at Q2 = 0.1 GeV2gluon & quark-antiquark sea production begins at Q2 = 5 GeV2
valence quark contributions [to nucleon momentum] are [swamped by continuum [sea] excitations at Q2 = 100 GeV2
(pg. 195)
u & d [valence] quarks dominate nucleon momentum at the lowest energy collisionsquarks emit gluons at higher energy transfers
more gluons & quark-antiquark pairs are produced at still higher energies
at the highest energies (~100 GeV), an equilibrium is reached where the nucleon momentum shared amongst all types of quarks & gluons equally
(pg. 196-7)
exotic (s,c) quarks are always produced in the sea [by gluon bond breaking (?)]below the charm quark production threshold, roughly half of the nucleon's momentum is carried by gluons
More specifically, the nucleon's momentum budget breaks down as:
5%antiquarks*(all in the sea)45%quarks50%gluons[electrically neutral material unaffected byEletro-Weakinteractions](pg. 231-34; 242-43, 256)
* Antiquarks are only produced in quark/anti-quark pairs. Therefore, these figures seemingly suggest, that 10% of the nucleon's momentum comes from broken gluon bonds (quark/antiquark pairs), 50% from gluons, and 40% from the three (3) main Valence [or "structural"] quarks. So, glue mass dominates sea quarks, even as it may dominate the masses of valence quarks (m0 = 4-5 MeV, meff = 330 MeV)
The sea is an SU(N) singlet [spin zero, Quantum Entangled (?)] state(pg. 247)
0 -
Have scientists seen "bare" exotic quarks (s, c, b, t), or do they only appear in Mesons & Baryons ?
0 -
According to The Teaching Company [Great Courses] Impossible: Physics beyond the Edge (lecture 21), the Quantum No-Cloning Theorem denies the possibility of "perfect" copying of quantum states. So, what is the "best" quantum copying which can be created ? Could you "quasi-clone" a quantum system with, say, 90% fidelity ?
0 -
According to The Teaching Company's [Great Courses] Impossible: Physics beyond the Edge (lecture 22), Electro-Magnetic Fields "enforce" Electric Charge conservation; and, Gravitational Forces "enforce" Mass-Energy conservation.
Does this mean, that Color Charge conservation is "enforced" by the Strong Force ???
0 -
From analyzing the aforecited figure, it looks like, compared to those of up quarks, the Wave Functions, of down quarks, are more "squeezed", into intermediate radii. To wit, the positive charge distributions of up quarks dominate, even relative to their greater electrical charges, in the inner core, and outer limbs, of the nucleons.
Uploaded with0 -
Not to my knowledge, which was why I'm confused. Frank Close's The New Cosmic Onion explicitly states that the charge of the proton is concentrated inside 3 distinct quarks, embedded in "electrically neutral material", thought to be the gluon field "glue".
Merged post follows:
Consecutive posts mergedIn D.Griffiths' Intro. to Elementary Particles (2nd ed.), quark Wave Functions are often modeled using standard, electronic, Hydrogen Atom Wave Functions -- the same also "recycled" for modeling Positronium.
Thus, maybe quarks are "small" (10-18 m) in the same sense as electrons are always said to be "small" (10-18 m) (as seen here), even tho their WFs are much larger (10-18 m for quarks, 10-10 m for electrons).
0 -
The "(pseudo)scalar" (S=0) mesons, [math]\pi^0 = (u \bar{u} - d \bar{d})/ \sqrt{2}[/math] and [math]\eta = (u \bar{u} + d \bar{d} - 2 s \bar{s})/ \sqrt{6}, \eta^{'} = (u \bar{u} + d \bar{d} + s \bar{s})/ \sqrt{3}[/math] contain contributions from d,u,s type quarks, in a Spin anti-parallel configuration. Thus, with their Spins separated by 1 unit, the individual quarks comprising those mesons could (hypothetically) emit, absorb, & exchange W-Bosons.
Is it possible, that the reason why those mesons are not "pure" [math]d \bar{d}, u \bar{u}, s \bar{s}[/math] states, but rather admixtures of them, is b/c the individual quarks comprising those mesons are (actually) undergoing Weak Force interactions, passing W-Bosons back & forth, and Flavour Oscillating ([math]d (W^{-} W^{+}) \bar{d} \to u (W^{+} W^{-}) \bar{u} \to s (W^{-} W^{+}) \bar{s} \to u (W^{+} W^{-}) \bar{u}[/math])... ???
0 -
Most comets may be from other solar systems | COSMOS magazine
Most comets may be from other solar systemsComputer simulations have revealed that famous long-period comets such as Halle-bop (pictured) are likely to have originated in another solar system...
more than 90% of comets in the Oort Cloud were captured from other stars when the Sun spread apart from its ‘birth cluster’. Levinson said it is common for stars to be born in ‘clusters’, fed by a large cloud of gas held together by its own gravity. Around each of these stars will form comets, many of which will be stripped from the star by the gravity of the cluster.
When some of the stars grow old enough, they emit strong stellar winds that literally blow the gas from the cluster, destroying its gravitational hold on the stars. Once this occurs the cluster starts to disperse, and if a star leaves the cluster at the same velocity as a comet from another star, the comet can be captured.
Trillions of comets on average originally form around each star, and the study’s computer simulations show up to a quarter of this average can end up in an Oort Cloud.
The fact the Sun stole so many comets doesn’t mean other stars missed out on the chance to have an Oort Cloud of their own. “It’s not a zero sum game,” said Levison, and noted for all of the comets captured by stars when the cluster dispersed, there are many times more floating around in inter-stellar space.
0 -
(Virtual) photons mediate all Electromagnetic phenomena, including the "Electrostatic Coulomb force". That's what confuses me -- how can two, static, point charges, each carrying opposite "signs" of electrical charge, attract each other, by exchanging (virtual) photons ??
Explaining how two point charges, each carrying the same "sign" of electrical charge, could repel each other, by exchanging (virtual) photons, is easy -- as Swansont pointed out, photons carry momentum, so that the two positive point charges, could "shoot" photons between themselves, and each time they "fired" a photon, they'd be "kicked backwards" (a little like a rocket), and each time they absorbed the other charge's photons, they'd be "kicked backwards" again. In such a way, two same-signed charges would repel each other.
But, how can you use photons, to explain Electrostatic attraction ?
0 -
According to Fundamentals in nuclear physics: from nuclear structure to cosmology, by Jean-Louis Basdevant, James Rich, Michel Spiro (pg. 156), the Electric Charge distributions, of nucleons, are essentially spherically symmetric:
Uploaded withWhere is the evidence of 3 quarks, in the aforegoing figures ?
0 -
Simple Model of Quarks inside Nucleons
Neglects rapidly rising Strong Force Potential at "rim" of nucleon, as well as Spin (& Orbital ???) angular momentum. Electrostatic Binding Energy of neutron is predicted to be about -1/3 MeV more negative than that for proton.
Uploaded withNucleon Clocks ???
Immediately after a Down quark decays into an Up, the supposed spatial arrangement of the quarks (dud --> duu) is "out of balance", compared to the stable state for protons (duu --> udu). Perhaps it takes some time for the "newborn" perturbed proton to "settle down" into said stable state ??
Could detailed scattering experiments observe this "settling down" process, which perhaps proceeds according to some sort of "decay half-life", as the "slightly excited" proton "leaks" away a little more energy (p* --> p) ???
Merged post follows:
Consecutive posts mergedMagnetic effects 25x less
The effect of colour combined with thePauli Exclusion Principleis that any twoquarkshaving the same flavour (twoup, twodown, twostrange) in the lowest energy state must spin parallel -- precisely the opposite of what happens in atoms & nuclei... If two flavours are identical, and the third differs (e.g., [math]\Delta^{++}[/math] (uud) orp (uud)), then the identical pair must spin parallel, but the third is not constrained it can spin parallel (hence, total spin 3/2, as in the [math]\Delta[/math]) or antiparallel (hence, total spin 1/2, as in the proton).Frank Close.The New Cosmic Onion, pg. 95.Uploaded withMerged post follows:
Consecutive posts mergedHigh-energy Electrons, scattered off of nucleons, react according to how many Quarks they encounter (??)
Frank Close.The New Cosmic Onion, pg. 88.Frank Close.The New Cosmic Onion, pg. 104.[math]\lambda_e = \frac{\hbar c}{\sqrt{E^2 - (m c^2)^2}} \approx \frac{\lambda_c}{\gamma}[/math](from)1 GeVElectronsface a "phalanx" ofQuarks, seeing a "shield wall" ofQuarks"wading" or "swimming" thruGluons. But, higher-energyElectronscan "hammer" onindividualQuarks,isolatedfrom their "phalanx formation". LoneQuarksare increasingly easy to "push around".Merged post follows:
Consecutive posts mergedNucleons are "3 quarks swimming in slime [= glue]"
It is analogous to quarks being held to one another by an elastic band that is slack. The quarks are apparently free, but after being struck, they recoil, and the elastic becomes tighter, preventing them from escaping. The elastic may become so stretched, that it snaps. The two new ends created have quarks on them, and so mesons are formed, but not free quarks...QCD predicts the following scenario, and experiment is consistent with it. Immediately after their production, the quark & antiquark move off in opposite directions. Initially, they feel no force, but as they separate, the energy in the force field between them grows, eventually becoming so great that its energy "E" exceeds the "mc2" needed to create further quarks & antiquarks. The initial quark & antiquark cluster together with these spontaneously created ones so quickly, forming mesons & baryons, that the original quark & antiquark are not detected; instead, two oppositely directed showers of hadrons emerge...
QCD predicts that, at high energies, the quark & antiquark usually carry off most of the energy, with the glue collimated along their direction of motion, carrying little energy itself. In such circumstances, two distinct jets of particles emerge.
Frank Close. The New Cosmic Onion, pp. 89-89,100-101.
David Griffiths.Introduction to Elementary Particles, pg. 72.Snapped "slime strings" bloop back into balls of slimy glue, imparting momentum (w/o carrying any momentum away)Merged post follows:
Consecutive posts mergedHooke's Potential (1/2 k x2) approximation
[math]1 GeV \approx \frac{1}{2} k r_0^2[/math][math]k \approx 10^{20} N/m[/math]When a quark strays 1 fm from its fellows, the force from this formula is 105 N -- to wit, 10 tons of force, acting across 1 fm, on 1 quark's mass.
This amounts to more than a billion billion trillion G's.
Quarks are rather rugged.
Merged post follows:
Consecutive posts merged"Slime ball" model = "Bag Model"
From Hyperphysics:
Thequarksof aprotonare free to move within the proton volumeIf you try to pull one of thequarksout, the energy required is on the order of1 GeV per fermi, like stretching an elastic bag.The energy required to produce a separation far exceeds the pair production energy of aquark-antiquarkpair, so instead of pulling out an isolatedquark, you producemesonsas the producedquark-antiquarkpairs combine.http://hyperphysics.phy-astr.gsu.edu/hbase/particles/quark.html#c6
http://hyperphysics.phy-astr.gsu.edu/hbase/nuclear/scatele.html#c1
Merged post follows:
Consecutive posts mergedPerhaps quarks in "glue" could be modeled as point masses in Visco-elastic fluid ??
Merged post follows:
Consecutive posts mergedMagnetic moment effects are PHENOMENAL
Assuming Quark masses, of 2.4 Mev (Up) & 4.8 Mev (Down), this simple model suggests that the Magnetic binding energies, of nucleons, are over 1 GeV, with the neutron being about 370 MeV heavier (less bound). A characteristic Magnetic Binding Energy:
[math]U_0 \equiv \frac{\mu_0 \mu_q^2}{4 \pi r_0^3} \approx 390 MeV[/math]compares favorably, with the mass difference (~300 MeV), between protons (ground state: all 3 quarks' magnetic moments aligned), and the [math]\Delta^{+}[/math] (excited spin-state: all 3 quarks' spins aligned). Thus, although not particularly precise, this simple model strongly suggests, that magnetic moment effects can account for the mass differences between baryons.
Further, from the "snapping slime-string" model mentioned above (PPs), that the [math]\Delta^{+}[/math] decays by emitting pions might mean, that gluon bonds break when "twisted a half-turn" (180 degrees), as the "middle" quark's spin flips back to anti-parallel (i.e., given its opposite charge, as its magnetic moment flips back to parallel). If so, "slime" might not be the best mental model of the "consistency" of gluon glue -- it might be more like Earth's semi-plastic Mantle magma.
Finally, the best-fit models, for the Potential Wells confining quarks, predict forces of order 900 MeV fm-1, or about 16 tons of force, at 1 fm, on each quark (D.Griffiths. Intro. Elementary Particles (2nd ed.), pg. 173) ! And, the magnetic moment binding energies, suggested by this simple model, are also right around 1 GeV fm-1. Thus, perhaps a "Magnetic Well" (~r-3) might poignantly approximate the potential keeping quarks confined ??
Merged post follows:
Consecutive posts mergedThe Neutral Rho Meson ([math]\rho^0[/math]) is an excited spin-state of the Neutral Pi-Meson / Pion ([math]\pi^0[/math]), where both quarks' mechanical Spins are parallel (making their Magnetic Moments anti-parallel, b/c of the opposite charge on the anti-quark). This "magnetic mis-alignment" makes the mass of the particle increase from ~135 MeV to 775 MeV, or by 640 MeV.
This is of the same order of magnitude, as the ~300 MeV mass increase caused by the spin-excitation of a proton into a [math]\Delta^{+}[/math]. This would seem to suggest, that even this simple model, accurately captures "the gist" of the mighty Magnetic Moment interactions amongst quarks, in hadrons. If anything, the numbers might mean, that the quarks in mesons orbit a bit closer together, by an amount (given the r-3 dependency of the Magnetic Moment energy) of order (640 MeV / 300 MeV)1/3 [math]\approx[/math] 1.3.
It does not seem implausible, that 2-quark mesons might be somewhat smaller than 3-quark baryons. And, if mesons were somewhat smaller spatially, that could account for their being allot less "glue" in mesons vs. baryons.
Merged post follows:
Consecutive posts mergedThe 'MIT Bag Model' [suggests that] free quarks of mass m, confined within a spherical shell of radius R, are found to have an effective mass [math]m_{eff} = \sqrt{m^2 + (\hbar x)/(R c)^2}[/math], where x is a dimensionless number around 2.5. Using the radius of the proton (say, 1.5 x 10-13 cm) for R, we obtain meff = 330 MeV / c2 for the up & down quarks.D.Griffiths. Intro. to Elementary Particles (2nd ed.), pg. 151.
Since, even for mesons, meff >> m, we find from that formula that [math]R \approx \frac{\hbar x}{c \, m_{eff}}[/math]. And, since, in mesons, meff is roughly 5x less, Rmeson is roughly 5x more -- to wit, ~7.5 fm.
Note, too, that, since gluons carry Color Charge, and so can interact with other gluons, one could conceivably claim that baryons have 6x more "glue" (see following figure) than mesons -- which would account for the fact that nucleons have ~6x more "glue mass" than pions (~930 MeV : ~130 MeV).
Uploaded withMerged post follows:
Consecutive posts mergedOpposite electric charges means that mechanical Spins parallel produce Magnetic Moments anti-parallel, et vice versa. Thus, for the ground states of nucleons (S = 1/2, n/p), that the oppositely charged quarks also Spin in opposite directions, means that all 3 quarks' Magnetic Moments are always co-aligned.
Thus, this simple picture predicts, that the Magnetic Moments, of protons vs. neutrons, should stand in the ratio of:
[math]\mu_p : \mu_n = \frac{+1 + \frac{1}{4} +1}{- \frac{1}{4} - 1 - \frac{1}{4} } = \frac{9/4}{-6/4} = -\frac{3}{2}[/math]in close agreement with the actual value of the ratio (-1.46).
Moreover, the neutron magnetic moment = -1.91 [math]\mu_N[/math], where [math]\mu_N[/math] is the Nuclear Magneton,
[math]\mu_N = \frac{e \hbar}{2 m_p} \approx 5.05 \; 10^{-27} J T^{-1}[/math]Thus, incorporating the constants from my model, w.h.t.:
[math]-1.91 \mu_N = - \frac{6}{4} \mu_u[/math]so that:
[math]\mu_u \equiv \frac{2/3 e \hbar}{2 m_u} = (2/3) (-1.91) \mu_N[/math]from which it becomes quite clear, that the numbers work out exactly, were one to set mu = mp / 1.91 = 490 MeV, which (for such a simple model) is surprisingly close to the actual mu,eff = 340 MeV. Of course, if one must use the effective mass, in all of the Magnetic Moment formulae, then magnetic effects are, indeed, "25x less" than the electrostatic effects, as originally claimed. But, again against that, is the fact that the excited spin-states of protons ([math]\Delta^{+}[/math]) and neutral pions ([math]\rho^0[/math]) are 300-600 MeV more massive, a "phenomenal" effect.
Merged post follows:
Consecutive posts mergedQuark effective masses reduce Magnetic Moments
Based upon Wikipedia's List of Baryons & List of Mesons, I compiled the following figures, for (1) the Effective Masses, of the various flavors of quarks, when bound in Baryons & Mesons; and (2) the energy cost, for flipping the spins of quarks, in Baryons & Mesons. All such values are in MeV.
Flavor Bare Mass Effective Mass (baryons) (mesons) Spin Flip Cost (baryons) (mesons) u 2 312 68 295 640 d 5 315 71 295 640 s 95 485 425 220 400 c 1300 1660 1800 65 140 b 4200 4990 5210 20 45 t 174000
Method:
For example, u+d quarks, in nucleons, must mass about 1/3 of that of their nucleon (~940 MeV), implying an meff of ~310 MeV per quark. Likewise, u+d quarks, in pions, must mass about 1/2 of that of their pion (~140 MeV), implying an meff of ~70 MeV per quark. Then, effective masses for the further flavors were estimated, successively, by "subtracting off" those of the flavors estimated already.
Spin-flip energy costs were calculated, in turn, by comparing excited spin states (S=3/2) with their ground-states (S=1/2). For instance, the [math]\Delta^{+}[/math] and [math]\Delta^0[/math] are the excited spin-states of the proton & neutron, each massing about 295 MeV more than their nucleon counterparts. Likewise, the [math]\rho^{+}[/math] and [math]\rho^0[/math] are the excited spin-states of the pions, each massing about 640 MeV more than their pion counterparts. Care, for the more massive quarks, was taken to try to select excited spin-states, where it was clear, which quark was flipped.
Results:
The product:
[math]m_{eff} \times SpinFlipCost \approx constant[/math]for baryons (but not mesons):
Flavor Meff * SFC (baryons) (mesons) u 92040 43520 d 92925 45440 s 106700 170000 c 107900 252000 b 99800 234450 t
This makes perfect sense. For, the energy cost, of flipping a Magnetic Moment, in a magnetic field, is [math]\Delta U = \mu B[/math], and quarks' Magnetic Moments are reduced by their effective masses:
[math]\mu_q = \frac{\hbar e_q}{2 m_{q,eff}}[/math]Merged post follows:
Consecutive posts mergedApproximating Nucleons as uniformly Magnetized w/ uniform internal Magnetic Field
From the formula for Magnetic Dipoles, w.h.t.:
[math]B(0) = \frac{2 \mu_0}{3} m \delta^3(\vec{r})[/math][math]m = k_{p,n} \mu_N[/math][math]k_p = +2.82, k_n = -1.91[/math][math]\delta^3(\vec{r}) \to \left( \frac{4 \pi r_0^3}{3} \right)^{-1}[/math][math]r_0 \approx 1.4 \; fm[/math][math]B(0) = k_{p,n} \frac{2 \mu_0}{4 \pi r_0^3} \mu_N \approx k_{p,n} \; 368 \; GT[/math][math]\mu_q \equiv \kappa_{u,d} \frac{\hbar e/3}{2 m_p/3} = \kappa_{u,d} \mu_N[/math][math]\kappa_u = 2, \kappa_d = -1[/math][math]\Delta U = 2 \times \mu_q B(0) = 2 \kappa_{u,d} k_{p,n} \frac{2 \mu_0}{4 \pi r_0^3} \mu_N^2[/math]To flip the Spin of the d in a p+ ([math]\to \Delta^{+}[/math]) therefore requires:
[math]2 \times 1 \times 2.82 \frac{2 \mu_0}{4 \pi r_0^3} \mu_N^2 \approx 65 KeV[/math]or 4 MeV were one to use the Bare Mass of the d in the determining its Magnetic Moment. And to flip the Spin of the u in a n0 ([math]\to \Delta^0[/math]) therefore requires:
[math]2 \times 2 \times 1.91 \frac{2 \mu_0}{4 \pi r_0^3} \mu_N^2 \approx 88 KeV[/math]or 14 MeV were one to use the Bare Mass of the u in determining its Magnetic Moment.
Merged post follows:
Consecutive posts mergedThe Magnetic Moments, of Deuterium & Tritium, appear to be (basically) simple sums, of the Magnetic Moments of protons (+2.82 [math]\mu_N[/math]) and neutrons (-1.91 [math]\mu_N[/math]):
particle Magnetic Moment (10[sup]-27[/sup] J/T) proton +14.106067 neutron -9.66236 deuteron +4.3307346 (= p+n) triton +15.046094 (= p+n-n)
This seemingly says, that in Deuterium (S=1), the proton & neutron are Spin-aligned (so that their Magnetic Moments add); and, in Tritium (S=1/2), the two neutrons are Spin-anti-aligned (so that their Magnetic Moments cancel out).
INTERPRETATION:
The Magnetic Moments, of nuclear particles, can be constructed, as simple sums, of the Magnetic Moments of their 'sub-particle' constituents.
APPLICATION:
The Magnetic Moments, of protons & neutrons, can be constructed, as simple sums, of the Magnetic Moments of their up & down quarks:
[math]\mu_p = + 2 \mu_u + \mu_d = +2.82 \mu_N[/math][math]\mu_d = - \mu_u - 2 \mu_d = -1.91 \mu_N[/math]Note that, in the ground states (S=1/2), of protons & neutrons, the mechanical Spins, of the oppositely charged quarks, are oppositely aligned ([math]p (u \uparrow u \uparrow d \downarrow), n (u \uparrow d \downarrow d \downarrow)[/math]), so that their Magnetic Moments are actually all aligned.
The solution, to this simple system of equations, is:
[math]\left( \stackrel{2}{1} \stackrel{1}{2} \right) \left( \stackrel{\mu_u}{\mu_d} \right) = \mu_N \left( \stackrel{+2.82}{+1.91} \right)[/math][math]\left( \stackrel{\mu_u}{\mu_d} \right) = \frac{\mu_N}{3} \left( \stackrel{2}{-1} \stackrel{-1}{2} \right) \approx \mu_N \left( \stackrel{5/4}{1/3} \right)[/math]Assuming that [math]\mu_q = \hbar e_q / 2 m_{q,eff} \times S[/math], with S=1/2, this strongly suggests, that the Effective Masses, of the up & down quarks, are roughly mp/4 & mp/2.
Merged post follows:
Consecutive posts mergedThe Magnetic Moments, of Deuterium & Tritium, appear to be (basically) simple sums, of the Magnetic Moments of protons (+2.82 [math]\mu_N[/math]) and neutrons (-1.91 [math]\mu_N[/math]):
particle Magnetic Moment (10[sup]-27[/sup] J/T) proton +14.106067 neutron -9.66236 deuteron +4.3307346 (= p+n) triton +15.046094 (= p+n-n)
This seemingly says, that in Deuterium (S=1), the proton & neutron are Spin-aligned (so that their Magnetic Moments add); and, in Tritium (S=1/2), the two neutrons are Spin-anti-aligned (so that their Magnetic Moments cancel out).
INTERPRETATION:
The Magnetic Moments, of nuclear particles, can be constructed, as simple sums, of the Magnetic Moments of their 'sub-particle' constituents.
APPLICATION:
The Magnetic Moments, of protons & neutrons, can be constructed, as simple sums, of the Magnetic Moments of their up & down quarks:
[math]\mu_p = + 2 \mu_u + \mu_d = +2.82 \mu_N[/math][math]\mu_d = - \mu_u - 2 \mu_d = -1.91 \mu_N[/math]Note that, in the ground states (S=1/2), of protons & neutrons, the mechanical Spins, of the oppositely charged quarks, are oppositely aligned ([math]p (u \uparrow u \uparrow d \downarrow), n (u \uparrow d \downarrow d \downarrow)[/math]), so that their Magnetic Moments are actually all aligned.
The solution, to this simple system of equations, is:
[math]\left( \stackrel{2}{1} \stackrel{1}{2} \right) \left( \stackrel{\mu_u}{\mu_d} \right) = \mu_N \left( \stackrel{+2.82}{+1.91} \right)[/math][math]\left( \stackrel{\mu_u}{\mu_d} \right) = \frac{\mu_N}{3} \left( \stackrel{2}{-1} \stackrel{-1}{2} \right) \approx \mu_N \left( \stackrel{5/4}{1/3} \right)[/math]Assuming that [math]\mu_q = \hbar e_q / 2 m_{q,eff} \times S[/math], with S=1/2, this strongly suggests, that the Effective Masses, of the up & down quarks, are roughly mp/4 & mp/2.
0
Irreversibility of "Measurement" ?
in Quantum Theory
Posted
The calculation of entropy, from "counting microstates", weights every such state, by its probability, yes? If so, the entropy of a|A> + b|B> is constant, for all a,b s.t. a+b = constant.
Now, when Wave Functions (WFs), of quantum objects, interact "weakly" (when it's "business as usual" under "normal & ordinary" kinds of conditions, such that the SWE still applies, without WF collapse), their WFs become "entangled".
So, strongly seemingly, you're quite correct -- [math]\Psi = c_1 (v_1 \otimes u_1) + c_2 (v_2 \otimes u_2) \rightarrow v_1 \otimes u_1[/math] (or, [math]v_2 \otimes u_2[/math]) conserves entropy.
What if particle number is not conserved, in the interaction, which causes WFC ? Please ponder a standard double-slit experiment, in which a detector screen (D) is irradiated by an incident electron beam (e-), in what amounts to a "reverse photoelectric effect":
where the ejected photon is rapidly reabsorbed, within the detector (D), as thermal radiation [the incident electron beam heats up the detector screen]. At deepest root, this rather resembles the WF "localization", of when a formerly free electron becomes bound to a bare proton during Recombination in the ISM or IGM. The SWE is completely "probability preserving", so the irreversible act of particle creation / destruction, in which a whole new probability distribution is "born", or an old probability distribution "vanishes", cannot mathematically be described with said SWE. This is quite akin to WFC, which also represents a "discontinuous quantum jump", beyond the scope or purview of the SWE. So, could not the "quantum measurement problem", of what causes WFC, have something to do, with particle number non-conservation ? And, would not a particle number increase, in an interaction, increase the ensemble's entropy (a whole new particle, with new states available to it, in addition to those available to the previous particles...) ?