
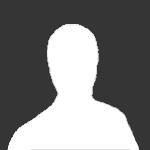
Widdekind
-
Posts
1121 -
Joined
-
Last visited
Content Type
Profiles
Forums
Events
Posts posted by Widdekind
-
-
OBSERVATION:
The Relative Raleigh Convection Score of Venus (M ~ 0.8, g ~ 0.9) is only 0.66. Thus, even "Earth's twin", being only slightly smaller, already drops down to ~2/3rds of the "convective vigour" of Earth.
According to Wikipedia, Plate Tectonics on Venus ended between 1,200 to 500 million years ago. Therefore, Venusian Plate Tectonics lasted for 3.4 to 4.1 billion years, from the formation of the Solar System. If Terrestrial Plate Tectonics last 3/2 as long, we should expect them to cease after 5-6 billion years (~0.5 to 1.5 billion years from now).
ANSWERING QUESTION:
I thought the general consensus was that Uranus and Neptune were worlds of extremely hot water oceans thousands of miles deep over Earth sized rocky cores. Far to hot to contain ice of any kind.Uranus' "Solid Core" is composed of Rocks (~Silicates) & Ices (~Water). Uranus' "Solid Core" is differentiated, into chemically distinct layers, analogous to the Core & Mantle of the Earth. And, even as Earth's Core & Mantle are quite hot, so, too, is the central "Solid Core" of Uranus. Thus, the Ices melt, forming a vast High-Pressure Ocean (even as Earth's Inner Core is liquid, albeit, liquid Iron -- at high-pressure, to wit, a "High-Pressure Iron Ocean").
Never-the-less, the above figure strongly suggests that the bulks of Uranus & Neptune are comprised of Rocks & Ices, w/ comparatively thin Atmospheric Envelopes.
Earth is a Rocky World, w/ merely a salting of Ices. Uranus & Neptune are Rock-Ice Worlds, having Rocks & Ices in nearly equal measures.
Uranus is made up of a swirl of gases, liquids, and solids. It has no solid surface to stand on. The solids have settled to the center of the planet. These include silicon, iron, and a basalt like rock. The core of Uranus is about the size of Earth. At the outer edge of the core... is an ocean that is about 5,000 miles deep. It is thought to be mostly water and a mixture of liquid ammonia and methane. An interesting thing about Uranus' ocean, is that it is extremely hot-almost 8,000 degrees Fahrenheit. At the surface of this ocean, there is a gaseous layer that is 4,000 to 5,000 miles deep. This layer of atmosphere is mostly liquid hydrogen, methane and some helium. The methane gas gives Uranus its blue-green color. Above the gas layer is another layer made of hydrogen and helium.Most planets orbit the sun by rotating and creating days and nights as Earth does. Uranus does not. Instead, its poles are on its sides. The planet spins from north to south instead of west to east. It takes it 17.25 hours to spin around completely. It is tilted so far on its side that it actually lies almost level with its orbital path around the sun. Most planets axis' tilt less than 30 degrees, but Uranus tilts at 98 degrees.
Traveling on an ovular path around the sun, it speeds through space at 4.25 miles per second. It takes 84 years for it to complete one orbit or one Uranus year. The mass of this planet is 14.5 times larger than that of Earth. Its density is about 1.27 grams per cubic centimeter [cf. Water ~ 1, Rock ~ 3] and the force of gravity is ninety percent of that on Earth. So if one were to weigh 100 pounds on Earth, he would weigh only 90 pounds on Uranus.
The atmosphere is composed of many gases. It is 83% hydrogen, 15% helium, 2% methane, and it has small amounts of ethane as well as other gases. The temperature of Uranus [Atmosphere] is about –355 degrees Fahrenheit. The interior temperature can rise fast and even reach up to 4,200 degrees in the ocean and 12,600 degrees at the core.
Merged post follows:
Consecutive posts mergedThis Journal Article deals explicitly with this topic.
0 -
Exoplanets are not Brown Dwarfs
1. Star Formation
Stars form within Giant Molecular Clouds (GMCs). These dark clouds block the light from background stars, thereby becoming black patches in the night sky*. Now,
Dark clouds spin slowly. Molecules of gas & dust inside the clouds bump around, just as they do in Earth's atmosphere.Deep inside these clouds, regions calledCoresform.Coresspin, just like the dark clouds that surround them.ACoreis the densest part of a cloud, but still far less dense than air**.And, it is within these Cores, or "Star Factories", that "most stars are born, within groups of hundreds or thousands of stars"***.
*Ray Jayawardhana.Star Factories, pg. 5. William Herschel, and his sister Caroline(18th Century AD), called these dark nebulae "holes in the heavens".**ibid, pg. 18.***ibid., pg. 24.Now, newborn stars are often surrounded by Proto-Planetary Disks of gas & dust:
The dust in aDiskabsorbs light from the star inside theDisk. The dust warms a little, and the warm dust glows atInfraredwavelengths.Astronomerscan detect theInfrared Emissionsthat come from the dust. A star w/ aDisklooks brighter inInfraredlight than a similar star w/o aDisk...In theStar Factoriesthat have been observed, at least half of all theone-million-year-oldstars emit Infrared light we can measure. That may mean thatabout half of all young stars haveDisks*.Each of these Disks "has more than ten times the mass" of our own Sun's Planetary System** -- to wit, over 5000 Earth-masses (MEarth) of material, as compared to some 500 MEarth of material, mostly made up by Jupiter (318 MEarth) & Saturn (96 MEarth), in our own Planetary System. Thus, "young Disks have huge amounts of gas & dust" (~1 Myr), while the Disks of adolescent stars have "much less gas & dust" (~10 Myr), and the Disks of mature stars have "only a small amount of gas & dust" (~100 Myr)***. This is probably b/c "young stars often produce strong [stellar] Winds", which gradually drive away Disk materials#. Indeed, our own Sun's Proto-Planetary Disk probably originally contained some 6000 MEarth of gas & dust##.
*ibid., pp. 27-28.**ibid., pg. 29.***ibid., pp. 43,32.#ibid., pg. 46 ;cf.##C.A.Scharf.Extrasolar Planets & Astrobiology, pp. 101-102. B/c "the planets represent material retained in theInner Solar Systemfollowing the heating up & eventual ignition of the proto-Sun", the observed amount of material making up our planets is "biased towards metals, since lighter elements will be lost". Therefore, this figure is deduced by adding back enoughHydrogen & Heliumgas, to bring theSolar Systemback up to primordialSolar Element Abundance Ratios. Thus,Planetary Formationin ourSolar Systemwas probably only about 10% efficient.B/c of the particular physics of Core Collapses, in GMCs, only stellar-mass objects (M > 0.063 MSun) can be created*.
*Scharf,ibid., pp. 50-52.Cf. Jeans Mass.2. Big Planets are not small Stars
Based upon observations of companion objects to Solar-type stars, "there are very few objects in the 'Brown Dwarf desert' between 0.01 and 0.08 solar masses". Indeed, objects possessing "a mass of the order of 0.08 times that of the Sun (about 80 times the mass of Jupiter)" generate enough internal heat & pressure to fuse Hydrogen into Helium, and become Hydrogen-burning Red Dwarfs. But, "if a body has less than thirteen times the mass of Jupiter (about 0.01 of the mass of the Sun)", then it cannot generate enough internal heat & pressure even to fuse Heavy Hydrogen (Deuterium), and "will therefore remain a planet". So,
This clear separation of stars[M > 0.08 MSun]and planets[M < 0.01 MSun]reminds us that the two types of bodiy are formed in different ways -- stars via gravitational collapse [in GMCCores], and planets by accretion of matter onto a rocky embryo w/in a[Proto-Planetary] Diskof gas & dust*.Note that the biggest observed Exoplanets (M ~ 13 MJupiter) contain around 4000 MEarth of material. Thus, the biggest possible planets gobble up essentially all of their parent stars' Disk materials (~5000 MEarth of material; see above). This clear constraint surely explains the observed lack of supra-planet, sub-stellar mass objects (to wit, Brown Dwarfs).
*F.Casoli & T.Encrenaz.The New Worlds, pp. 61-63.0 -
so a planet made of exactly the same elements [as] Mars in the same proportions would be dense as the earth if it was much bigger than mars?
Yes -- the rock would compress under its own Self Gravity. Look at Earth itself -- our Iron Core has a density of ~11, yet, Iron on the Surface only has a denisty of ~8. Why is core Iron so much more dense? B/c it's compressed, by incomprehensible pressures.
The Moon, Mars, Venus, and Earth are all of (basically) comparable compositions -- b/c they all formed in the same liquid water "Climate Zone" around the Sun -- but their Bulk (Average) Densities increase in the same order as their Masses, and Self-Gravities.
Mercury is comparatively over-dense... but it formed so close to the Sun, that liquid water turned to steam vapor. Thus, Mercury lacks allot of Volatiles compared to the other Rocky Planets, so that it is composed of greater concentrations of heavy, dense elements like Nickel & Iron.
In the other direction, the Gas Giants formed out past where liquid water turns to ice. This increased the density of solid materials in the Proto-Solar Disk, allowing the Gas Giant proto-planets to get much bigger -- big enough to keep their Primordial H/He Atmospheres.
To brazenly coin terms, then, the Sun is surrounded by "Climate Zones" -- "steam zone" (Mercury), "water zone" (Venus, Earth, Moon, Mars), "ice zone" (Jupiter, Saturn Gas Giants), "methane-ice zone" (Uranus, Neptune Ice Giants). Within a single such "Climate Zone", the planets all tend to have similar overall average Bulk Compositions. And, all common materials compress under applied pressures.
Merged post follows:
Consecutive posts mergedTANGENT: Looking again at the attached figure, it seems that Uranus & Neptune are only slightly larger, in Radius, than purely solid Pluto-like Ice Planets of the same Mass. This suggests that Uranus & Nepture are "almost" giant Plutos -- to wit, that their Atmospheres are relatively thin, and that "most" of those Ice Giants are actually solid Icy Cores. Conversely, Jupiter & Saturn are accurately described by the Gaseous H/He curve, suggesting that they are, in fact, true Gas Giants possessing only comparatively small solid cores.
1 -
The Inner Solar System is depleted in both Hydrogen*, and Carbon**. The chemical combination of Hydrogen & Carbon, Methane (CH4), was a principal component of Earth's Primordial Volcanic Degassings (~4.5 to 4.4 Ga)***. And, in the Proto-Solar Nebula, Methane Ices only froze-formed beyond about 30 AU#. So, surely, Methane only existed as a Gas, in the Inner Solar System.
*W.T. Sullivan III & J.A. Baross.Planets and Life, pg. 88.See also:Attachment 1**ibid., pg. 463.See also:Attachment 2***ibid., pg. 101.#Carroll & Ostlie.Introduction to Modern Astrophysics(1996 ed.), pg. 893.CONCLUSION (?):
During the Sun's T-Tauri Phase, for roughly the first 10 million years of its existence, "the gas & dust of the Proto-Planetary Disk were expelled... all that remained were the Protoplanets, or the largest Embryonic Planets"*. Perhaps the young Sun's vigorous Solar Wind expelled copious quantities of Methane Gas, from the Inner Solar System, simultaneously explaining the depletions of Hydrogen & Carbon from that region.
*F.Casoli & T.Encrenaz.The New Worlds, pg. 72.0 -
Judging from my attached figure (from Carroll & Ostlie), such a large-but-low-density Planet would probably be composed of Ices. To wit, it would be a "Super Pluto".
Perhaps, then, you could have Convection Currents of water, or various Ices. To wit, like Europa. But, according to Eq. 2 ([math]Ra \propto M \; g^{2}[/math]), Europa's Raleigh Convection Number is 0.00015, roughly 1% of that of Mars.
0 -
1. Simple Model of Terrestrial-Planetary Structure
Advanced Computer Simulations of Planetary Structures give the relationship, between Planet Radius vs. Planet Mass, for Terrestrial Planets*. For Terrestrial Planets, of sufficiently small Planetary Mass (M [math]\leq 10 \; M_{\oplus}[/math]), this result can be linearly approximated accurately. For such Rocky Worlds, of Earth-like Composition, the Average Bulk Density ([math]\rho[/math]) increases nearly linearly with Surface Gravity (g):
[math]\rho = \rho_{0} + k \times g[/math](eq. 1)where, using Earth-normalized units:
[math]\rho_{0} \approx 0.536[/math][ 2.96 g cm-3][math]k \approx 0.464[/math][ 1 - p0 ]Note that [math]\rho_{0}[/math] represents the natural "uncompressed density", of Terrestrial-type rocks, at zero Surface Gravity, consistent with the observed density of Asteroids**.
*Carroll & Ostlie.Introduction to Modern Astrophysics(1996 ed.), pg. 825.See also:Attachment 1.**Carroll & Ostlie.ibid., pg. 878.AsteroidIda has a density of 2.2 - 2.9 g cm-3. Our "uncompressed density" is slightly higher, as we are here dealing with solid bodies, whereasAsteroidsare comparatively uncompactedRubble Piles. See also:2. Estimation of Terrestrial-Planetary Raleigh Convection Numbers
Earth's Plate Tectonics are driven by massive Convective Currents in the Earth's Mantle*. Such Convective Systems are characterized by the dimensionless quantity called the Raleigh Convection Number (Ra)**, defined as:
[math]Ra \equiv \frac{\Delta \rho \; g \; L^{3}}{D \; \mu}[/math]where:
[math]\Delta \rho[/math] is the density contrast across the convective regiong is the local gravitational accelerationL is the characteristic length-scale of convectionD is theDiffusivity[math]\mu[/math] is theDynamic ViscosityThe Dynamic Viscosity ([math]\mu[/math]), being complicated function of Temperature, will be discussed in an Appendix to this post. The Diffusivity (D) is a function of the Thermal Conductivity & Specific Heat Capacity of the convecting material, assumed to be constant for Terrestrial-type Bulk Compositions, and inversely proportional to the density of said material***.
*History ChannelHow the Earth was Made -- The Alps[TV]*****Thus, for our model of Planetary Convection, for a presumed planet of density [math]\rho[/math], radius R, Surface Gravity g, and mass M, we adopt:
[math]D \propto \rho^{-1}[/math][math]L \propto R[/math][math]\Delta \rho \equiv \rho - \rho_{0} = k \times g[/math]so that:
[math]Ra \propto \rho \; \Delta \rho \; g \; R^{3} \propto \rho \; g^{2} \; R^{3} \propto M \; g^{2}[/math]where we have used the basic identity that [math]M = \rho \times R^{3}[/math] (Earth-normalized units). Or, noting that [math]g = \rho \times R[/math] (Earth-normalized units), we have that:
[math]Ra \propto \frac{g^{5}}{\rho^{2}} = \frac{g^{5}}{\left( \rho_{0} + k \times g \right)^{2}} \approx 4 \; \frac{g^{5}}{\left( 1 + g \right)^{2}}[/math](eq. 2)where we have observed that both [math]\rho_{0}[/math] and k are each roughly equal to one-half*.
*M is cubic in g, making impossible a simple closed-form solution, for Ra, in terms of M alone.3. Analysis
Eq. 2 is a steeply increasing function of Surface Gravity. For Mars (M = 0.1, g = 0.4), [math]Ra \approx 0.016[/math] (Earth-normalized units). Thus, Mars' lower density ([math]\rho[/math]), lower density contrast ([math]\Delta \rho[/math]), lower Surface Gravity (g), and smaller size ®, all combine to make Mars two Orders-of-Magnitude less convective than Earth. This is consistent with the observed lack of Plate Tectonics upon that planet.
Note that a hypothetical Super-Earth ([math]g = 2, M \approx 3.6[/math]) would have a Raleigh Convection Number of [math]Ra \approx 14[/math]. Thus, such Super-Earths could quite conceivably be much more convective than Earth.
0 -
Computer Simulations suggest that:
hundreds of medium-sizedBlack Holesare roaming loose in theMilky Way. These rogues... are theorphanedCentral Black Holesof the many smallerGalaxiesthat theMilky Wayhas swallowedover itsbillions of yearsof existence.For:
Galaxies, such as our own, most often grow by absorbing smaller, satellite galaxies, such as theLarge Magellanic Cloud, which orbits theMilky Way.And:
each of the hundreds of billions ofGalaxiesin theCosmosformed a massive or supermassiveBlack Holeat its center [and] when bigGalaxiescollide -- a relatively common occurrence -- their centralBlack Holeseventually merge.But:
sometimes the centralBlack Holeof aDwarf Galaxymight remain independent after aGalactic Collision... The ejectedBlack Holewould not move fast enough to escape theGalaxy'sgravity entirely, but it would move faster than the background stars--something that makes it detectable, because it would also be dragging along asmall cluster of surrounding stars... the researchers suggest searching the galaxy for compact and relatively fast-movingclusters of old stars*.Now, "compact clusters of old stars" sounds suspiciously like Globular Clusters:
supermassiveGlobular Clustersare in fact the cores ofDwarf Galaxiesthat are consumed by the largerGalaxies.SeveralGlobular Clusters(likeM15[in ourMilky Way Galaxy,33.6 thousand light-years away, inPegasus Constellation])have extremely massive Cores which may harborBlack Holes...a4,000 Solar-massIntermediate-mass Black Holehas been suggested to exist, based on HST[Hubble Space Telescope]observations,in theGlobular ClusterM15, and a20,000 Solar-massBlack Holein theMayall IIcluster in theAndromeda Galaxy. Both x-ray and radio emissions from Mayall II appear to be consistent with an intermediate-mass black hole.These are of particular interest because they are the first black holes discovered that were intermediate in mass between the conventional stellar-massBlack Holeand the supermassiveBlack Holesdiscovered at the cores ofGalaxies.The mass of theseIntermediate-mass Black Holesis proportional to the mass of the clusters, following a pattern previously discovered betweenSupermassive Black Holesand their surroundingGalaxies**.This strongly suggests, that Globular Clusters are ancient Dwarf Galaxies, having "Dwarf Black Holes" at their cores.
***0 -
Back in the 1960s AD, Geologists thought that deep ocean Life was impossible, down in the Abyssal blacknesses beneath the surface Photic Zone, where Photosynthesis is no longer possible. But, the discovery of Hydro-Thermal Vent systems, along the Mid-Ocean Ridges, by Bob Ballard and others, proved that Chemosynthetic Life thrives, even w/o Sunlight*.
*National Geographic ChannelNaked Science -- Dangers of the Deep(TV)Now, free-floating, inter-stellar, Rogue Planets could conceivably be quite common, for example, in the Orion Nebula*. Large Rogue Planets could probably keep thick Atmospheres, which would insulate them against the cold blackness of space. Beneath such a blanket, Geothermal processes could keep the planet warm enough for water. However, they would "be difficult to detect due, to the intrinsically weak Thermal Microwave Radiation emissions emanating from the lower reaches of the Atmosphere"**. Note that most Rogue Planets are probably sub-stellar-mass "failed stars", as opposed to "ejected planets"***.
***,***CONCLUSION (??): The "Predator Homeworld" may be a large Rogue Planet, supporting a complex, and exclusively Chemosynthetic, Ecology, including an advanced Extra-Terrestrial Intelligence (ETI) "Predator".
0 -
In the spirit of Stephen Webb's book, If the Universe Is Teeming with Aliens ... Where Is Everybody?: Fifty Solutions to the Fermi Paradox and the Problem of Extraterrestrial Life, this author offers:
Solution 51 -- Aliens are Predators
According to Webb (p.113),
One reason why ETCs[Extra-Terrestrial Civilizations]might choose to keep quiet isfear. When an ETC broadcasts to space, it reveals its location, and perhaps level of technology. Any neighbors who are listening may beaggressive...Perhaps caution is a general trait among advanced intelligences.I offer that this is due to Natural Selection -- the Milky Way Galaxy is populated by an advanced Predatory Civilization, that has already annihilated -- to wit, "predated" -- all the unwary intelligences in our Galaxy.
Indeed, Webb proposes basically this hypothesis, but uses the word "Berserker" instead of "Predator" (p. 112). By but replacing these two words, in Webb's proposal, we have that:
ETCs have either been prevented from arising by[Predators], wiped out by[Predators], or else are keeping quiet for fear of attracting[Predators]... So, why have[Predators]silenced all otherCivilizations, but left us alone? We could argue that[Predators]destroy onlyTechnological Life-forms, and need a "trigger" -- presumably the detection ofRadio Waves-- before they begin to work.Note that yet another of Webb's proposals is called "They are here, and are meddling in Human affairs". This "Berserker-Predator" hypothesis succinctly sets a pre-Industrial Glass Ceiling upon emerging Civilizations, neatly explaining the apparent lack of the same in our skies.
0 -
In the spirit of Stephen Webb's book, If the Universe Is Teeming with Aliens ... Where Is Everybody?: Fifty Solutions to the Fermi Paradox and the Problem of Extraterrestrial Life, this author offers:
Solution 51 -- Aliens are Predators
According to Webb (p.113),
One reason why ETCs[Extra-Terrestrial Civilizations]might choose to keep quiet isfear. When an ETC broadcasts to space, it reveals its location, and perhaps level of technology. Any neighbors who are listening may beaggressive...Perhaps caution is a general trait among advanced intelligences.I offer that this is due to Natural Selection -- the Milky Way Galaxy is populated by an advanced Predatory Civilization, that has already annihilated -- to wit, "predated" -- all the unwary intelligences in our Galaxy.
Indeed, Webb proposes basically this hypothesis, but uses the word "Berserker" instead of "Predator" (p. 112). By but replacing these two words, in Webb's proposal, we have that:
ETCs have either been prevented from arising by[Predators], wiped out by[Predators], or else are keeping quiet for fear of attracting[Predators]... So, why have[Predators]silenced all otherCivilizations, but left us alone? We could argue that[Predators]destroy onlyTechnological Life-forms, and need a "trigger" -- presumably the detection ofRadio Waves-- before they begin to work.Note that yet another of Webb's proposals is called "They are here, and are meddling in Human affairs". This "Berserker-Predator" hypothesis succinctly sets a pre-Industrial Glass Ceiling upon emerging Civilizations, neatly explaining the apparent lack of the same in our skies.
0 -
-
Stellar Habitable Zones are defined by:
L*/DHZ2~constAnd, stellar Luminosities scale approximately as the fourth-power of the star Mass (Bowers & Deeming. Astrophysics I: Stars, pg. ~28).
Thus, the Habitable Zones of smaller & dimmer stars are deeper down into their parent stars' Gravity Wells:
UHZ=- G M*/ DHZ~M*-1And so, Interplanetary Space Travel in such systems would require considerably greater (~several times more) Energy expenditures.
Moreover, most M-Class stars' potentially Habitable Planets, b/c they orbit so closely to their parent star, are probably Tidally Locked to those stars*, and probably possess no Moons**. Thus, those potentially Habitable Planets will spin much more slowly, making Rocket Launches even more Energetically costly, and they will possess no nearby Planetoids for easily accessible off-world Colonies.
*BBC / Channel 4Alien Worlds(DVD)**Moreover still, M-Class stars are often violent Flare Stars*. Thus, Interplanetary Space Travel in such systems would pose greater Radiation Hazards to space-farers.
*BBC / Channel 4Alien Worlds(DVD)However, most M-Class stars' potentially Habitable Planets must, apparently, be comparatively small*. They would, thusly, have lower Surface Gravities, making them comparatively easier to escape.
***0 -
Crab Nebula (~10 light years across)
Figure 1.Crab Nebula(~10 light-yearsacross)Large Scale Structure (~109 light-years across)
Figure 2.Computer simulation ofLarge Scale Structure(~109light-yearsacross) ()Merged post follows:
Consecutive posts mergedCONCLUSION:
Gravitationally-induced Filament Formation in expanding blobs of gas & dust is common, across the Cosmos, across at least 9 Orders of Magnitude of spatial scale.
Merged post follows:
Consecutive posts mergedWILD SPECULATION:
Perhaps the Big Bang was the explosive evaporation of a Hyper-Massive Black Hole (HMBH), of hegemonically gargantuan scale. If so, the Big Bang was not a single, instantaneous event, but rather the culmination of a (very) protracted process. That is, the Big Bang would then be somewhat "smeared out", across many millions of years (Calculations to follow), as the HMBH progressively evaporated.
It might, then, even be possible to observe Gradients in the Cosmic Microwave Background, or other physical characteristics of the Cosmos, representing roughly the Radial Vector pointing black to the HMBH remnant. Surely, that remnant, akin to the Crab Nebula Pulsar, is not within our "Level-1 Parallel Univese*" -- to wit, not (yet) w/in our Visible Universe, being more than ~15 billion light-years away, and as yet Causally Disconnected -- for otherwise it would surely dominate the night- & day-time skies.
*History ChannelThe Universe -- Parallel Universes(TV)Merged post follows:
Consecutive posts mergedCalculations for Wild Speculations
As derived from Wikipedia, Black Holes, evaporating by Black Hole Radiation, decrease their mass as:
[math]M(t) = M_{0} \left( 1 - \frac{t}{\tau} \right)^{1/3}[/math]where:
[math]\tau = \frac{5120 \pi G^{2} M_{0}^{3}}{\hbar c^{4}} = 2.1 \times 10^{67} \left( \frac{M_{0}}{M_{\odot}} \right)^{3} years[/math]Since the Visible Universe has ~1025 (??) MSun of material, our hypothetical Hyper-Massive Black Hole (HMBH) would take ~10140 years to evaporate. Thus, the Big Bang would have been "spread out" over a time-scale of that order of magnitude, literally Googles & Googles of years.
0 -
Hi Widdekind!
I am glad you came back and checked your thread. From some other discussion at some other board, I forget what the topic was, I connect you with UCSD.
Maybe you did some of your undergrad or graduate work there. If so you may have run into Tytler? I don't know him, just of him. He works with Keck and he seems to be interested in the habitable planet search. Maybe directly involved in it.
I think the habitable planet search is exciting, actually in part because it spurs the development of new instruments.
And possibly will also spur the development of new methods of inference and mathematical applications (I don't know about this but it could be.)
Who else is good in that line of research? Any people or projects I should keep an eye out for? Don't know much about it, just feel it has potential.
Small world, I TA'd for Prof. Tytler's course about basic cosmology. As for other, University of WA Prof. Woodrow Sullivan is involved in Astrobiology. U-WA also has the Virtual Planetary Laboratory (VPL).
QUICK RE-EXPLANATION for CLARITY:
Habitable Planets presumably have copious quantities of water, which is necessary for Life -- to wit, they have Oceans.
Oceans moderate surface temperatures. For example, the waters off the coast of Chile stay about 55 degrees F year-around*. Likewise, coastal lands have much more mild climates than places far inland (eg. Gobi Desert, Siberia)**.
*History ChannelHow the Earth was Made -- Driest Place on Earth(TV)**National Geographic ChannelNaked Science -- Death of the Earth(TV)Thus, to make an analogy w/ food...
You can eat 2,000 calories every day...
Or, you can 20,000 calories one day, and fast for the next 9.
Likewise, an Eccentric planet can absorb huge amounts of energy at Perihelion, close in to the central star...
warm way up...
and then gradually cool back down, as it swings back out to Apihelion, far away from the central star.
Since the Oceans moderate the surface temperatures, heat re-radiation, back out to space, won't change that much, as the planet orbits. Thus, to "First Approximation", the planet's Black Body Radiation is constant, and so all that matters, for Thermal Equilibrium, is the Time Averaged Heat Influx, from the Sun, averaged over an orbital cycle.
So, w/ you representing our hypothetical Habitable Planet, "you" could "eat" 2,000 "calories" per "month", for 12 months...
Or, you could eat 3,000 "calories" for one month, 2,000 "calories" for 10 months, and then 1,000 "calories" for another month.
Overall, on average, that's still a "healthy diet". Sort of like Bears, hibernating for winter (0 calories eaten per day), after gorging themselves all summer (zillions of calories eaten per day). Overall, on average, the "Bears" remain lean & healthy.
These approximations would break down for highly Eccentric orbits (e ~ 0.8, say). But, as already noted above, of all ~300 planets known, nearly all have e < 0.2-0.3. In such a case, my simple model is valid, b/c it only makes a few percent difference, and is negligible anyway.
So, there's really no argument, but Eccentricity is still something to bear in mind, when considering Habitabilities of Planets.
Note: It's somewhat more complicated, b/c Eccentric orbits must have larger Semi-Major Axes, and hence longer years (Kepler's 3rd Law). So, a more accurate analogy would be:
-
eat 3,000 calories for one month
-
eat 2,000 calories for 10 months
-
eat 1,500 calories for 2 months
which still Averages out to 2,000 calories per month.
0 -
eat 3,000 calories for one month
-
That is a fair suggestion, I would "edit"
in the quotes if I could. I was indeed referring to "seasons" caused by high Eccentricty. However, the eccentricity term is, functionally, of the same form as the "Gamma Factor" in Special Relativity. The effect only becomes appreciable for very high eccentricities, e ~ 0.8+.
Never-the-less, Eccentricity seems worthy of consideration, for more complete pictures of Habitability issues.
(Carroll & Ostlie was the required text in Grad School, evidently being widely popular, w/ both Prof. and students.)
0 -
A star's Habitable Zone (HZ) is the region, around said star, where water exists as a liquid. In essence, the Habitable Zone is the Water Zone (Venus, Earth, Mars). Within this Water Zone, closer in towards the central star, water cannot condense. In essence, this is the Steam Zone (Mercury). But, beyond the Habitable Zone, farther from the central star, where water freezes, is the Ice Zone (Jupiter, Saturn)*. Roughly speaking the Habitable Zone is bounded between Rain Line (where water condenses as a liquid) and the Snow Line (where water freezes out as snow).
*Carroll & Ostlie.Introduction to Modern Astrophysics, pg. ~893. Even farther from the central star,Methanealso freezes, making aMethane Ice Zone(Uranus, Neptune).Now, by virtue of forming farther from their central stars, out in the Ice Zone, colder planets can keep more of their water, through their Planet Formation process. For example, Jupiter's small moon Europa has about ten times (10x) more water than the Earth*. It probably possesses a Global Ocean about 100 km deep, making the moon the Solar System's largest reservoir of liquid water**. Indeed, ice's condensation dramatically increases the density, in early circum-stellar disks, of solid material. This causes the accumulation of significantly larger Proto-Planetary Cores (~10 Mearth), which can then retain their primordial Gaseous Envelopes, thereby accounting for the Gas Giants' huge masses***.
*Prof. Woodruff T. Sullivan.Athena Lecture Series -- Astrobiology(Lecture #4),8 Mar. 2009 AD.**National Geographic ChannelNaked Science -- Journey to Jupiter(TV)***Caleb Scharf.Astrobiology & Extra-Solar Planets.Conversely, closer in towards the central star, ever hotter temperatures boil away increasing quantities of water, making such inner planets progressively drier (cf. Mercury). Thus, most water on such planets is probably brought back in by impacts, from Planetesimals, Comets, & Asteroids, scattered into the inner system, by the pull of Gas Giants' gravities (eg. Jupiter) from further out*.
*National Geographic ChannelNaked Science -- Birth of the Oceans(TV)CONCLUSIONS: For Habitable Zones, Primordial Water retention, through the Planet Forming process, is inversely proportional to Temperature, which increases inwards, towards the central star. Thus, all else being equal, potentially habitable planets' quantities of water should tend to decrease inwards, towards the central star. In particular, potentially habitable planets born on the outer edge of their parent stars' Habitable Zones could frequently be created possessing comparatively bigger planetary Oceans.
Furthermore, potentially habitable planets orbiting smaller, dimmer stars (M,K-Class) could get greater quantities of water, by being physically closer to their system's Snow Line and water-bearing Icy Bodies. This is because the physical width (in AU) of a star's Habitable Zone is proportional to that star's mass. To see this, first consider an orbiting planet's expected Black Body Temperature, at Thermal Equilibrium:
[math]\frac{L_{*}}{4 \pi r^{2}} \; ( \pi R_{p}^{2}) = \sigma T_{p}^{4} \; (4 \pi R_{p}^{2})[/math]Now, Planetary Global Temperatures (Tp) must be essentially the same, in all Habitable Zones (~300 K), by definition of liquid water being present. So, for a given star (L*), the Orbital Radius ® of the Habitable Zone is:
[math]r^{2} = \frac{L_{*}}{16 \pi \sigma T_{p}^{4}}[/math]Thus, the width of that star's Habitable Zone is roughly:
[math]\delta r = \sqrt{\frac{L_{*}}{16 \pi \sigma}} \left( \frac{-2}{T_{p}^{3}}\right) \delta T_{p} = -2 r \frac{\delta T_{p}}{T_{p}}[/math]Since, by definition, every Habitable Zone has essentially the same requisite Planetary Temperature (Tp ~ 300 K) and permissible temperature drop (dTp ~-100 K), the width of Habitable Zones scales as their radii.
In addition, stars' Luminosities (L*) roughly scale as the fourth power of their Masses (M*)*. So:
[math]\delta r \propto r \propto M_{*}^{2}[/math]Bigger stars become so much brighter, that their Habitable Zones are pushed profoundly further outwards, where the Temperature Gradient (~r-1/2) is significantly lower, which widens the Habitable Zone.
*Bowers & Deeming.Astrophysics I -- Stars, pg. ~26.Thus, the distance between a potentially habitable planet, orbiting in its parent star's Habitable Zone, and that star system's Snow Line and water-bearing Icy Bodies beyond, is dramatically reduced around smaller & dimmer stars. This could conceivably make Water Transport, by those water-bearing bodies, more common, creating larger Oceans, on habitable planets in such systems.
ADDENDUM: It can be shown, that an orbiting planet's Total Energy (Ep) and Angular Momentum (Lp) are roughly:
[math]E_{p,tot} = - \frac{G M_{*}}{2 r}[/math][math]L_{p} = \sqrt{G M_{*} r (1-e^{2})}[/math]Restricting our attention to Habitable Zones, where dr ~ r ~ M*2, we therefore have that (as above):
[math]\delta E_{p,tot} = - E_{p,tot} \frac{\delta r}{r} \propto M_{*}^{-1}[/math][math]\delta L_{p} = \frac{L_{p}}{2} \frac{\delta r}{r} \propto M_{*}^{3/2}[/math]Thus, compared to Habitable Zone planets orbiting bigger & brighter stars, those orbiting around smaller & dimmer stars are:
-
Physically closer, in terms of Orbital Radius, to their star system's Snow Line & Icy Bodies
-
More similar, in terms of Angular Momentum, to those Icy Bodies
-
More different, in terms of Total Energy, from those Icy Bodies
Thus, if differences in Total Energy dramatically reduce the gravitational scatterings, of Icy Bodies into Inner Systems, Habitable Zone planets orbiting smaller & dimmer stars could conceivably tend to be comparatively Water Poor. But, if differences in Angular Momentum, or Orbital Distance, are the biggest barriers to Water Transport, Habitable Zone planets orbiting smaller & dimmer stars could conceivably tend to be comparatively Water Rich.
0 -
Physically closer, in terms of Orbital Radius, to their star system's Snow Line & Icy Bodies
-
Please ponder a potentially Habitable Planet, complete w/ Atmosphere & Oceans sufficient to support possible Life. Now, imagine that this planet's orbit is notably Eccentric. Thus, this planet dives down in, near to its parent star, rapidly absorbing copious quantities of energy (surely, a short Summer). Then, the planet pulls back out, far from its parent star, and gradually cools (long Winter).
SPECULATION: The parent star's Habitable Zone (HZ) basically comprises that region, wherein that star's observed Energy Flux (Watts per area) is comparable to that of our Sun, at our Earth (~1400 W / m2).
But, b/c a potentially habitable planet can absorb energy, into its Oceans, we can consider an averaged Energy Flux, over one full revolution of the planet (to wit, one Orbital Year):
[math]Time\;Averaged\;Enegy\;Flux \equiv \frac{1}{Orbital Period} \int Energy Flux \; \partial{t}[/math]But:
[math]Energy\;Flux = \frac{L_{*}}{4 \pi r^{2}}[/math]And (?):
[math]\partial{t} = \frac{\partial{\theta}}{\frac{\partial{\theta}}{\partial{t}}} = \frac{\partial{\theta}}{\dot{\theta}}[/math]So:
[math]\overline{\Phi} \equiv \frac{1}{P} \int_{0}^{2 \pi} \frac{L_{*}}{4 \pi r^{2}} \frac{\partial{\theta}}{\dot{\theta}}[/math]Now, it is well-known, that Planetary Orbits conserve (Specific) Angular-Momentum:
[math]\widetilde{\ell} \equiv \widetilde{r} \times \widetilde{v} = Constant[/math]And so we can consider (using well-known Vector Identities):
[math]\ell^{2} = \left( \widetilde{r} \times \widetilde{v} \right) \bullet \left( \widetilde{r} \times \widetilde{v} \right) = \left( \widetilde{r} \bullet \widetilde{r} \right)\left( \widetilde{v} \bullet \widetilde{v} \right) - \left(\widetilde{r}\bullet\widetilde{v}\right)\left(\widetilde{r}\bullet\widetilde{v}\right)=\left(r^{2}\right)\left(v^{2}\right)-\left(r\dot{r}\right)\left(r\dot{r}\right)[/math]where we have decomposed the Orbital Velocity (v) (technically, the velocity of the Reduced Mass, of the Reduced One-Body Problem) as:
[math]\vec{v} = \dot{r} \; \hat{r} + r \; \dot{\theta} \; \hat{\theta}[/math]So:
[math]v^{2} = \vec{v} \bullet \vec{v} = \dot{r}^{2} + r^{2} \; \dot{\theta}^{2}[/math]and:
[math]\ell^{2} = \left( r^{2} \right) \left( v^{2} - \dot{r}^{2}\right) = r^{4} \; \dot{\theta}^{2} [/math]Taking the square-root, and plugging back in above, we have that:
[math]\overline{\Phi} \equiv \frac{1}{P} \int_{0}^{2 \pi} \frac{L_{*}}{4 \pi \ell} \partial{\theta} = \frac{L_{*}}{2 P \ell} [/math]Now, it can be shown (see below post) that:
[math]\ell = \sqrt{G M_{*} a (1-e^{2})}[/math]and:
[math]P^{2} = a^{3} \frac{4 \pi^{2}}{G M_{*}}[/math]Therefore, we have that:
[math]\overline{\Phi} = \frac{L_{*}}{4 \pi a^{2}} \frac{1}{\sqrt{1-e^{2}}} [/math]CONCLUSION: As a potentially habitable planet's Eccentricity (e) increases, its Semi-Major Axis (a) must also increase, to keep constant its annual energy intake. This derivation assumes that the habitable planet's Global Temperature, being moderated by its Oceans, does not change considerably over the course of its orbit, and that, thusly, neither does its thermal re-radition rate. This effect is minute for most Eccentricities.
0 -
Thanks for the EvoEdu link !
0
Why Mars has no Plate Tectonics ?
in Astronomy and Cosmology
Posted
"Rock" is the "frozen ice" form of Magma.
Likewise, "Ice" is the frozen form of Water.
So, just as Earth is a "Rocky World", even though its interior is molten Magma...
so, too, Uranus is a "Rock-Ice World", even though its interior is molten Magmas & liquid Water.
B/c of Uranus' super-thick, "ultra-Venusian" Atmosphere, its "Surface Temperature", down in at the base of its Atmosphere, is probably so high that the whole "Ice Mantle" is "molten" (ie, that it's a High-Pressure, High-Temperature, liquid water ocean).
As an analogy, consider Venus. Imagine that Venus' Atmosphere was even thicker, and its Green-House Effect even higher. You could imagine, then, that such a "super Venus" would not have a solid Rocky Crust... but, be covered entirely in a molten Magma Ocean (like the early Earth). Now, just replace the word "Rocky" for "Icy" -- the "surface" of Uranus is a "molten Ice (= water) ocean".
Ice is to Water
as
Rock is to Magma.
Earth's interior is melted Rock...
Uranus' interior is melted Ice