
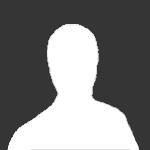
Science Student
-
Posts
71 -
Joined
-
Last visited
Content Type
Profiles
Forums
Events
Posts posted by Science Student
-
-
This is a bit counter-intuitive, I suppose. It puzzled me when I was young.
One thing to note is that we are dealing with pressure. This is force (weight) per unit area. So the area is already (implicitly) taken into account. If you expressed the equation terms of force (not pressure) then you would need to include area in the equation. But then you could simplify it by replacing force/are with pressure. Back to square 1! (And, actually, I'm not sure how you would get force, other than multiplying pressure by area. And then the area would just cancel out.)
There is something deeply wrong with how I am trying to understand this. I know that the surface of the water exposed to the atmosphere doesn't matter because I have read it in multiple sources. But I don't understand why.
When I look at the picture that you posted at the top, I see that there is a certain amount of surface area of water in the petri dish that is exposed to atmospheric pressure. So, the equation pressure = force/area leads me to believe that force = area*pressure which seems to mean that the more surface there is the more force will be applied to the water.
Or as an example, if I apply x pressure to a 2m^2 side of a rock, then the force applied = 2m^2*x. But if I apply x pressure to a different side of the rock that is 3m^2, the force is greater f = 3m^2*x; this might move the rock where the other force might not.
This is driving me crazy because I know I am wrong, but I feel like I am right.
0 -
It is because water can pour out of the bottom and let air in. If the tube is narrow enough, then there will be sufficient surface tension to stop this happening. I have no idea know how one would work that out, but it may involve surface area...
Oh I see.
What about the size of the petri dish; do you think that the more surface for the atmosphere to push on, the more water weight that can be held up in the down glass upside down?
0 -
Actually, you seem to have that the wrong way round. Atmospheric pressure would keep the water in the cup ... until the cup got really big! It is easier to test this by putting a filled bottle under water and slowly pulling it out (with the opening under the water). You can see that the water stays in the bottle.
The pressure caused by a column of water is density x height x g. If this pressure is greater than air pressure then it will no longer be supported by the atmosphere and you will get a gap at the top (which will actually be a vacuum). This requires a tube about 10 metres high so tricky to do in practice. Easier with mercury, which only requires a height of about 1 m.
I have read what you have in your second paragraph before, but I don't understand it.
I understand that the atmospheric pressure acts on the surface of the water in the petri dish. So then why would water come out of an open test tube if the test tube was not submerged in the water of the petri dish? Does it have something to do with the surface of the water that the atmospheric pressure acts on? If so, then wouldn't this "10m rule" require a certain area of water that the atmospheric pressure must act on?
The equation that I was hoping for to make sense of all of this would have a water surface variable included.
0 -
As a thought experiment, I imagine having a full cup of water sealed with plastic saran wrap. I think that if I turned it upside down in a shallow puddle of water in a petri dish and slipped off the saran wrap, then the water would probably come out of the glass causing the petri dish to overflow.
So how much water do I need for the atmospheric pressure to be enough to keep the water in the glass.
0 -
It can — there's an infinite number of combinations. But you can only easily solve for ones where you know that one variable is held constant.
Okay, I think I am getting it.
Thanks everyone!
0 -
[math]\frac{V_1*p_1}{T}=\frac{V_2*p_2}{T}[/math]
When you will decrease volume (f.e. close one end syringe and push it), you will increase pressure inside.
But then why does the pressure change and not the temperature? Or why doesn't pressure and temperature change?
0 -
Damn, I did not explain the issue properly.
I should have asked how temperature can be a function of volume. In other words, how does decreasing the volume of a container increase the temperature of the gas? Or would it?
In terms of V1/T1 = V2/T2, I don't think my air compressor heated up when it would take in and compress the air. Although I may not have noticed.
0 -
I understand how pressure is a function of temperature, but not how temperature a function of pressure.
My notes have: initial volume / initial temperature = final volume / final temperature.
This seems to mean that adding pressure increases temperature. But how does pressure increase the average kinetic energy of the air molecules?
I can understand how increasing the average kinetic energy of air molecules increases the pressure on the walls of the container. But vice versa doesn't make sense to me.
0 -
Extracted from your link.
Yes that's exactly what I was trying to steer you towards.
There is not one factor involved but a combination of them.
The number of hydrogen atoms
The number of lone pairs
The geometry which is the result of hybridisation and allows attractions between lone pairs and the Hd+
all play their part.
Note that the 4 and 2 are the max numbers of hydrogen bonds, not all may be established.
Keep asking searching questions and you will go far. +1 for a good question
Thank-you, and thank-you for giving your opinion about the link; that is helpful too.
0 -
H2O
H1F
How many hydrogen bonds (involving hydrogen) can each molecule engage in?
I think the answer is 4 for water and 2 for hydrogen flouride
Note also that boiling point increases with increasing molecular weight, in the absence of molecular interactions.
Water is (16 + 2) = 18 : BP = 100C
Hydrogen Flouride is (19 + 1) = 20 : BP = 20C
Ammonia is (14 + 3) = 17 : BP = -33C
Methane is (12 + 4) = 16 : BP = -182C
So water and hydrogen flouride are anomalous, going across the periodic table, as are hydrogen sulphide and hydrogen chloride in the next line, for the same reasons.
Thanks for your help, but it's just that my textbook doesn't seem to get into enough details for this question. I found an answer that seems correct at http://www.chemguide.co.uk/atoms/bonding/hbond.html about a third of the way down.
0 -
So how many hydrogen bonds must be 'broken' to release a molecule from the liquid to the gas in each case?
Knowing the number of hydrogen bonds for these polar molecules is not something that is covered in the material before the question.
However, I see that there would probably be more hydrogen bonds in HF per molecule than H2O because there are more lone pairs on F for hydrogen from the other hydrogen fluorides to bond to. This makes HF's boiling point even more counterintuitive to me.
0 -
What are the shapes of the molecules and which ones have lone pairs?
HF has 3 lone pairs and H2O has 2 lone pairs. H2O is a bent tetrahedral, and HF has only a single bond, so I am not sure what to call it. This question is in my textbook for grade 11 chemistry; it wants me to know why, but I can't find anything in the chapter that explains it.
0 -
I checked the electronegativities of the intermolecular forces, and the difference between fluorine and hydrogen is 1.8, much higher than oxygen and hydrogen which is 1.2. So there must be another reason other than hydrogen bonding why H2O has such a higher boiling point than HF, which is only 19.5c.
Also, why isn't HF called hydrogen monoflouride?
1 -
Yes the book is unclear and you have correctly deduced that for a non spinning spherical Earth the weight would be the same at the poles and the equator.
For a more complete derivation you need to think in three dimensions.
Firstly the geometry.
In fig1 any point of the has latitude [math]\lambda [/math] on the surface of a sphere of radius R.
If we take horizontal (East-West) sections as shown by the dashed line through the point on the surface we generate a smaller circle of radius [math]{R_\lambda }[/math].
This has centre [math]{C_\lambda }[/math], as shown in fig4 beneath.
It is important to remember that the centripetal/centrifugal action takes place in this plane and is not directed towards the cente of the Earth.
This is shown in fig3
In fig2 we see that the acceelation due to gravity is directed towards the centre of the Earth.
This acceleration is the same everywhere on the surface of a rotating or non rotating spherical Earth.
The accelerations are vector quantities and combine vectorially so the component of the acceleration due to the rotation is
[math]{\omega ^2}{R_\lambda }\cos \lambda [/math]
ie
[math]{\omega ^2}\left( {R\cos \lambda } \right)\cos \lambda [/math]
Now the object of mass m is resting on the Earth's surface, but it is not in absolute equilibrium since it is spinning with the Earth.
We can reduce it to euqilibrium by introducing the fictious centrifugal force which acts outwards and opposes the force due to gravity or the weight of the mass.
This is shown in fig 6.
This centrifugal force reduces the weight of the mass by the equation shown at the bottom.
So the apparent weight as measured by any device that does not balance weights eg a spring balance will be lower than that measured by a weight balance eg a beam balance since the weights in the beam balance are affected the same as the mass.
Thank-you so much, I understand it much better now.
Thank-you everyone!!!!
0 -
I guess I am really just wanting to know why the textbook says that g is less on the equator rather than saying that g - ac is 0.03 m/s^2 less than g. Doesn't g stay the same no matter how fast the Earth spins?
0 -
There is wording in my textbook that is frustrating me, or it is me who does not understand the concept.
After explaining the centripetal acceleration at the equator but not at the poles, my textbook says, "for a spherically symmetric earth the acceleration due to gravity should be about 0.03 m/s^2 less at the equator than at the poles".
The textbook says it this way multiple times, so I know it wasn't a typo.
Shouldn't it be due to gravity and the centripetal acceleration? Or shouldn't it have said "due to the apparent g"?
0 -
If I'm understanding you correctly, then the short answer is "yes," the point of intersection will be "the same."
The longer answer is that while R2 is a subset of R3, vectors in R2 aren't really the same objects as vectors of the form <x, y, 0> in R3. However, R2 is isomorphic to (i.e. has the same structure as) the subspace of R3 containing these vectors, so results in one will "carry over" to the other.
I hope that explanation isn't too muddy.
That makes sense; thanks a lot!
0 -
The actual question implies that this is possible, but I am just curious how.
The rows in R2 are only in two dimensions. So geographically, this seems to mean that the point where the lines cross would still be in R2, right?
Or am I misunderstanding what it means to "be in R3"?
0 -
One way we might (begin to) construct the natural numbers is to define 0 as the empty set Ø and define the successor function S(x) = x ∪ {x} for any set x. It follows that each natural number n ≠ 0 equals {0, 1, 2, ..., n - 1}. So we have
0 = Ø
1 = S(0) = {0} = {Ø}
2 = S(1) = {0, 1} = {Ø, {Ø}}
3 = S(2) = {0, 1, 2} = {Ø, {Ø}, {Ø, {Ø}}}
etc. Of course, only heretics take zero to be a natural number, and this construction still works if we begin with 1 = Ø instead of 0 = Ø, but I think the latter is slightly easier to conceptualize.
In any case, we can then define the relation < in terms of elementhood such that a < b ↔ a ∈ b. Thus, from the construction given above, 1 < 2.
Of course, there are other possible constructions (though as far as I know, they all follow the same general process). A nice example, along with a more detailed definition and discussion of <, can be found here.
Oh god, this is well beyond what I was expecting - thanks a lot!
1 -
It's not possible for infinitely many physical things to make up a kilogram. If you take a kilogram of any material substance and subdivide it finely enough you'll end up with a large but finite number of subatomic particles. There are only finitely many quarks in the universe, far less than 10^100 in fact.
It's true that there are infinitely many points in a mathematical line segment, but that has nothing to do with the physical world.
But it might be true for energy or space-time. If we look at energy or space-time as continuous like the real number line, then maybe infinitesimal energies or infinitesimal distances apply. A physicist once told me that a Planck's constant is not necessarily the smallest possible quantity of energy, just the smallest detectable quantity of energy.
0 -
In other words, what axiom implies 1 < 2?
1 -
Your question brings up an interesting bit of history because Archimedes was the first to successfully study this question and he wrote down his mathematical treatment in the middle of the third century BC.
Unfortunately this document (called The Methodf) was lost in antiquity and only rediscovered in 1910, when and old parchment was cleaned.
Anyway suppose we consider this thought experiment:
Take a ruler and pencil and draw a thin line 25mm long.
Draw another line right along side the first line so that you cannot see any gaps between them.
Continue drawing lines for about three hours.
You will then have a rectangular area on your paper.
No imagine sharpening you pencil and repeating the experiment.
It will now take you many more lines to draw the same rectangle, say six hours work.
Sharpen again and repeat.
Perhaps you can see where this is going.
The thinner the line the more you need to create the area until.
Until the line is so thin the number is ver very large indeed.
This is what is meant by tending to infinity.
This was exactly the process by which Archimedes derived his famous mensuration formulae, and the process by which we add up a very large number of very small contributions to create a whole.
It is well known that we can add up an infinite number points to obtain a finite total.
Mathematically that is what taking limits is about.
However you ask how can an infinite number of points add up to different values?
Well the simplest way to see this is to look at and compare a couple of infinite series.
[math]\sum {_1^\infty \frac{1}{{{1^2}}} + \frac{1}{{{2^2}}} + \frac{1}{{{3^2}}} + \frac{1}{{{4^2}}} + \frac{1}{{{5^2}}}.............} [/math][math] = 1.645[/math]
[math]\sum {_1^\infty \frac{1}{{{1^3}}} + \frac{1}{{{2^3}}} + \frac{1}{{{3^3}}} + \frac{1}{{{4^3}}} + \frac{1}{{{5^3}}}.............} [/math][math] = 1.202[/math]
You can see by direct term by term comparison that these two series have the same (infinite) number of terms, but their sums to infinity are different.
Wow, that's so amazing!!!!
0 -
I am really confused over the general idea of the summation of infinitesimals of some quantity. For example, can anyone show me mathematically how an infinite number of dm can either equal 5 kg's or 10 kg's? I can understand basic calculus.
0 -
I get 0.00225 for the moment of inertia calculation
Oh thank-you so much!!!! I checked my work about 15 times and could not see that.
0
Is there an equation for how much water can rise in a vacuum?
in Applied Chemistry
Posted
Thanks Strange and everyone else, I get it now!